Actin filament turnover drives leading edge growth during myelin sheath formation in the central nervous system
- PMID: 26166299
- PMCID: PMC4736019
- DOI: 10.1016/j.devcel.2015.05.013
Actin filament turnover drives leading edge growth during myelin sheath formation in the central nervous system
Abstract
During CNS development, oligodendrocytes wrap their plasma membrane around axons to generate multilamellar myelin sheaths. To drive growth at the leading edge of myelin at the interface with the axon, mechanical forces are necessary, but the underlying mechanisms are not known. Using an interdisciplinary approach that combines morphological, genetic, and biophysical analyses, we identified a key role for actin filament network turnover in myelin growth. At the onset of myelin biogenesis, F-actin is redistributed to the leading edge, where its polymerization-based forces push out non-adhesive and motile protrusions. F-actin disassembly converts protrusions into sheets by reducing surface tension and in turn inducing membrane spreading and adhesion. We identified the actin depolymerizing factor ADF/cofilin1, which mediates high F-actin turnover rates, as an essential factor in this process. We propose that F-actin turnover is the driving force in myelin wrapping by regulating repetitive cycles of leading edge protrusion and spreading.
Copyright © 2015 Elsevier Inc. All rights reserved.
Figures
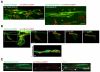
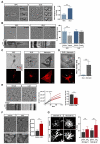
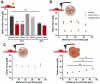
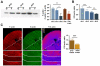
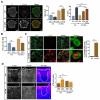
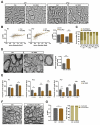
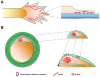
Similar articles
-
CNS myelin wrapping is driven by actin disassembly.Dev Cell. 2015 Jul 27;34(2):152-67. doi: 10.1016/j.devcel.2015.06.011. Epub 2015 Jul 9. Dev Cell. 2015. PMID: 26166300 Free PMC article.
-
Depressing time: Waiting, melancholia, and the psychoanalytic practice of care.In: Kirtsoglou E, Simpson B, editors. The Time of Anthropology: Studies of Contemporary Chronopolitics. Abingdon: Routledge; 2020. Chapter 5. In: Kirtsoglou E, Simpson B, editors. The Time of Anthropology: Studies of Contemporary Chronopolitics. Abingdon: Routledge; 2020. Chapter 5. PMID: 36137063 Free Books & Documents. Review.
-
Reduced cofilin activity as a mechanism contributing to endothelial cell stiffening in type 2 diabetes.Am J Physiol Heart Circ Physiol. 2025 Jan 1;328(1):H84-H92. doi: 10.1152/ajpheart.00667.2024. Epub 2024 Nov 29. Am J Physiol Heart Circ Physiol. 2025. PMID: 39611817
-
Myelin repair in vivo is increased by targeting oligodendrocyte precursor cells with nanoparticles encapsulating leukaemia inhibitory factor (LIF).Biomaterials. 2015 Jul;56:78-85. doi: 10.1016/j.biomaterials.2015.03.044. Epub 2015 Apr 15. Biomaterials. 2015. PMID: 25934281 Free PMC article.
-
Impact of residual disease as a prognostic factor for survival in women with advanced epithelial ovarian cancer after primary surgery.Cochrane Database Syst Rev. 2022 Sep 26;9(9):CD015048. doi: 10.1002/14651858.CD015048.pub2. Cochrane Database Syst Rev. 2022. PMID: 36161421 Free PMC article. Review.
Cited by
-
A Novel Lysolecithin Model for Visualizing Damage in vivo in the Larval Zebrafish Spinal Cord.Front Cell Dev Biol. 2021 May 20;9:654583. doi: 10.3389/fcell.2021.654583. eCollection 2021. Front Cell Dev Biol. 2021. PMID: 34095120 Free PMC article.
-
Knockdown of Unconventional Myosin ID Expression Induced Morphological Change in Oligodendrocytes.ASN Neuro. 2016 Sep 21;8(5):1759091416669609. doi: 10.1177/1759091416669609. Print 2016 Oct. ASN Neuro. 2016. PMID: 27655972 Free PMC article.
-
Intrinsic and extrinsic regulators of oligodendrocyte progenitor proliferation and differentiation.Semin Cell Dev Biol. 2021 Aug;116:16-24. doi: 10.1016/j.semcdb.2020.10.002. Epub 2020 Oct 22. Semin Cell Dev Biol. 2021. PMID: 34110985 Free PMC article.
-
Mechanotransduction assays for neural regeneration strategies: A focus on glial cells.APL Bioeng. 2021 Apr 30;5(2):021505. doi: 10.1063/5.0037814. eCollection 2021 Jun. APL Bioeng. 2021. PMID: 33948526 Free PMC article. Review.
-
The emerging role of galectins in (re)myelination and its potential for developing new approaches to treat multiple sclerosis.Cell Mol Life Sci. 2020 Apr;77(7):1289-1317. doi: 10.1007/s00018-019-03327-7. Epub 2019 Oct 18. Cell Mol Life Sci. 2020. PMID: 31628495 Free PMC article. Review.
References
-
- Aggarwal S, Yurlova L, Snaidero N, Reetz C, Frey S, Zimmermann J, Pahler G, Janshoff A, Friedrichs J, Muller DJ, et al. A Size Barrier Limits Protein Diffusion at the Cell Surface to Generate Lipid-Rich Myelin-Membrane Sheets. Dev Cell. 2011;21:445–456. - PubMed
-
- Bauer NG, Richter-Landsberg C, Ffrench-Constant C. Role of the oligodendroglial cytoskeleton in differentiation and myelination. Glia. 2009;57:1691–1705. - PubMed
-
- Benninger Y, Thurnherr T, Pereira JA, Krause S, Wu X, Chrostek-Grashoff A, Herzog D, Nave KA, Franklin RJ, Meijer D, et al. Essential and distinct roles for cdc42 and rac1 in the regulation of Schwann cell biology during peripheral nervous system development. J Cell Biol. 2007;177:1051–1061. - PMC - PubMed
Publication types
MeSH terms
Substances
Grants and funding
LinkOut - more resources
Full Text Sources
Other Literature Sources
Molecular Biology Databases
Research Materials