Phosphoproteomics reveals malaria parasite Protein Kinase G as a signalling hub regulating egress and invasion
- PMID: 26149123
- PMCID: PMC4507021
- DOI: 10.1038/ncomms8285
Phosphoproteomics reveals malaria parasite Protein Kinase G as a signalling hub regulating egress and invasion
Abstract
Our understanding of the key phosphorylation-dependent signalling pathways in the human malaria parasite, Plasmodium falciparum, remains rudimentary. Here we address this issue for the essential cGMP-dependent protein kinase, PfPKG. By employing chemical and genetic tools in combination with quantitative global phosphoproteomics, we identify the phosphorylation sites on 69 proteins that are direct or indirect cellular targets for PfPKG. These PfPKG targets include proteins involved in cell signalling, proteolysis, gene regulation, protein export and ion and protein transport, indicating that cGMP/PfPKG acts as a signalling hub that plays a central role in a number of core parasite processes. We also show that PfPKG activity is required for parasite invasion. This correlates with the finding that the calcium-dependent protein kinase, PfCDPK1, is phosphorylated by PfPKG, as are components of the actomyosin complex, providing mechanistic insight into the essential role of PfPKG in parasite egress and invasion.
Figures
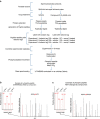
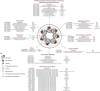
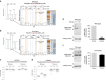
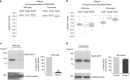
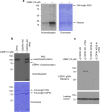
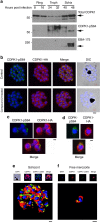
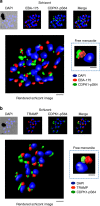
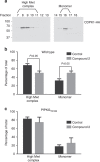
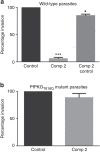
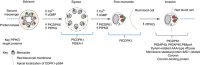
Similar articles
-
The malaria parasite cyclic GMP-dependent protein kinase plays a central role in blood-stage schizogony.Eukaryot Cell. 2010 Jan;9(1):37-45. doi: 10.1128/EC.00186-09. Epub 2009 Nov 13. Eukaryot Cell. 2010. PMID: 19915077 Free PMC article.
-
Mechanism of allosteric inhibition in the Plasmodium falciparum cGMP-dependent protein kinase.J Biol Chem. 2020 Jun 19;295(25):8480-8491. doi: 10.1074/jbc.RA120.013070. Epub 2020 Apr 21. J Biol Chem. 2020. PMID: 32317283 Free PMC article.
-
Plasmodium falciparum Cyclic GMP-Dependent Protein Kinase Interacts with a Subunit of the Parasite Proteasome.Infect Immun. 2018 Dec 19;87(1):e00523-18. doi: 10.1128/IAI.00523-18. Print 2019 Jan. Infect Immun. 2018. PMID: 30323024 Free PMC article.
-
The role of cGMP signalling in regulating life cycle progression of Plasmodium.Microbes Infect. 2012 Aug;14(10):831-7. doi: 10.1016/j.micinf.2012.04.011. Epub 2012 May 3. Microbes Infect. 2012. PMID: 22613210 Free PMC article. Review.
-
Extensive differential protein phosphorylation as intraerythrocytic Plasmodium falciparum schizonts develop into extracellular invasive merozoites.Proteomics. 2015 Aug;15(15):2716-29. doi: 10.1002/pmic.201400508. Epub 2015 May 19. Proteomics. 2015. PMID: 25886026 Review.
Cited by
-
Phosphorylation of Rhoptry Protein RhopH3 Is Critical for Host Cell Invasion by the Malaria Parasite.mBio. 2020 Oct 6;11(5):e00166-20. doi: 10.1128/mBio.00166-20. mBio. 2020. PMID: 33024030 Free PMC article.
-
Comparative Phosphoproteomic Analysis of Sporulated Oocysts and Tachyzoites of Toxoplasma gondii Reveals Stage-Specific Patterns.Molecules. 2022 Feb 2;27(3):1022. doi: 10.3390/molecules27031022. Molecules. 2022. PMID: 35164288 Free PMC article.
-
The Methods Employed in Mass Spectrometric Analysis of Posttranslational Modifications (PTMs) and Protein-Protein Interactions (PPIs).Adv Exp Med Biol. 2019;1140:169-198. doi: 10.1007/978-3-030-15950-4_10. Adv Exp Med Biol. 2019. PMID: 31347048 Free PMC article. Review.
-
Analysis of CDPK1 targets identifies a trafficking adaptor complex that regulates microneme exocytosis in Toxoplasma.bioRxiv [Preprint]. 2023 Aug 29:2023.01.11.523553. doi: 10.1101/2023.01.11.523553. bioRxiv. 2023. Update in: Elife. 2023 Nov 07;12:RP85654. doi: 10.7554/eLife.85654 PMID: 36712004 Free PMC article. Updated. Preprint.
-
Temporal and thermal profiling of the Toxoplasma proteome implicates parasite Protein Phosphatase 1 in the regulation of Ca2+-responsive pathways.Elife. 2022 Aug 17;11:e80336. doi: 10.7554/eLife.80336. Elife. 2022. PMID: 35976251 Free PMC article.
References
-
- Johnson L. N. Protein kinase inhibitors: contributions from structure to clinical compounds. Q. Rev. Biophys. 42, 1–40 (2009). - PubMed
-
- Lucet I. S., Tobin A., Drewry D., Wilks A. F. & Doerig C. Plasmodium kinases as targets for new-generation antimalarials. Future Med. Chem. 4, 2295–2310 (2012). - PubMed
Publication types
MeSH terms
Substances
Grants and funding
LinkOut - more resources
Full Text Sources
Other Literature Sources
Molecular Biology Databases