Reverse Transcription of Retroviruses and LTR Retrotransposons
- PMID: 26104704
- PMCID: PMC6775776
- DOI: 10.1128/microbiolspec.MDNA3-0027-2014
Reverse Transcription of Retroviruses and LTR Retrotransposons
Abstract
The enzyme reverse transcriptase (RT) was discovered in retroviruses almost 50 years ago. The demonstration that other types of viruses, and what are now called retrotransposons, also replicated using an enzyme that could copy RNA into DNA came a few years later. The intensity of the research in both the process of reverse transcription and the enzyme RT was greatly stimulated by the recognition, in the mid-1980s, that human immunodeficiency virus (HIV) was a retrovirus and by the fact that the first successful anti-HIV drug, azidothymidine (AZT), is a substrate for RT. Although AZT monotherapy is a thing of the past, the most commonly prescribed, and most successful, combination therapies still involve one or both of the two major classes of anti-RT drugs. Although the basic mechanics of reverse transcription were worked out many years ago, and the first high-resolution structures of HIV RT are now more than 20 years old, we still have much to learn, particularly about the roles played by the host and viral factors that make the process of reverse transcription much more efficient in the cell than in the test tube. Moreover, we are only now beginning to understand how various host factors that are part of the innate immunity system interact with the process of reverse transcription to protect the host-cell genome, the host cell, and the whole host, from retroviral infection, and from unwanted retrotransposition.
Figures
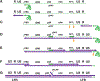
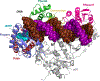
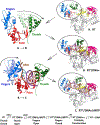
Similar articles
-
The diversity of retrotransposons and the properties of their reverse transcriptases.Virus Res. 2008 Jun;134(1-2):221-34. doi: 10.1016/j.virusres.2007.12.010. Epub 2008 Feb 7. Virus Res. 2008. PMID: 18261821 Free PMC article. Review.
-
Reverse transcription of retroviruses and LTR retrotransposons.Cell Mol Life Sci. 2001 Aug;58(9):1246-62. doi: 10.1007/PL00000937. Cell Mol Life Sci. 2001. PMID: 11577982 Free PMC article. Review.
-
Viral reverse transcriptases.Virus Res. 2017 Apr 15;234:153-176. doi: 10.1016/j.virusres.2016.12.019. Epub 2016 Dec 30. Virus Res. 2017. PMID: 28043823 Review.
-
The multiple roles of the nucleocapsid in retroviral RNA conversion into proviral DNA by reverse transcriptase.Biochem Soc Trans. 2016 Oct 15;44(5):1427-1440. doi: 10.1042/BST20160101-T. Biochem Soc Trans. 2016. PMID: 27911725 Review.
-
Retroviral reverse transcriptases.Cell Mol Life Sci. 2010 Aug;67(16):2717-47. doi: 10.1007/s00018-010-0346-2. Epub 2010 Apr 1. Cell Mol Life Sci. 2010. PMID: 20358252 Free PMC article. Review.
Cited by
-
Detection of feline immunodeficiency virus by neutral red-based loop-mediated isothermal amplification assay.Vet World. 2024 Jan;17(1):72-81. doi: 10.14202/vetworld.2024.72-81. Epub 2024 Jan 8. Vet World. 2024. PMID: 38406374 Free PMC article.
-
Nucleocapsid Protein Precursors NCp9 and NCp15 Suppress ATP-Mediated Rescue of AZT-Terminated Primers by HIV-1 Reverse Transcriptase.Antimicrob Agents Chemother. 2020 Sep 21;64(10):e00958-20. doi: 10.1128/AAC.00958-20. Print 2020 Sep 21. Antimicrob Agents Chemother. 2020. PMID: 32747359 Free PMC article.
-
HMMPolish: a coding region polishing tool for TGS-sequenced RNA viruses.Brief Bioinform. 2023 Sep 20;24(5):bbad264. doi: 10.1093/bib/bbad264. Brief Bioinform. 2023. PMID: 37478372 Free PMC article.
-
The Involvement of Ubiquitination and SUMOylation in Retroviruses Infection and Latency.Viruses. 2023 Apr 17;15(4):985. doi: 10.3390/v15040985. Viruses. 2023. PMID: 37112965 Free PMC article. Review.
-
Why the HIV Reservoir Never Runs Dry: Clonal Expansion and the Characteristics of HIV-Infected Cells Challenge Strategies to Cure and Control HIV Infection.Viruses. 2021 Dec 14;13(12):2512. doi: 10.3390/v13122512. Viruses. 2021. PMID: 34960781 Free PMC article. Review.
References
-
- Telesnitsky A, Goff GP. 1997. Reverse transcriptase and the generation of retroviral DNA, p. 121–160. In Coffin JM, Hughes SH, Varmus HE (ed), Retroviruses. Cold Spring Harbor Laboratory Press, Cold Spring Harbor, NY. - PubMed
-
- Hu W, Hughes SH. 2012. HIV-1 Reverse Transcription, p. 37–58. In Bushman FD, Nabel GJ, Swanstrom R (ed), HIV from Biology to Prevention and Treatment. Cold Spring Harbor Laboratory Press, Cold Spring Harbor, NY.
-
- Skalka AM, Goff SP. 1993. Reverse Transcriptase. Cold Spring Harbor Laboratory Press, Cold Spring Harbor, NY. - PubMed
-
- Gilboa E, Mitra SW, Goff S, Baltimore D. 1979. A detailed model of reverse transcription and tests of crucial aspects. Cell 18:93–100. - PubMed
Publication types
MeSH terms
Substances
Grants and funding
LinkOut - more resources
Full Text Sources
Miscellaneous