TORC2 mediates the heat stress response in Drosophila by promoting the formation of stress granules
- PMID: 26054799
- PMCID: PMC4510851
- DOI: 10.1242/jcs.168724
TORC2 mediates the heat stress response in Drosophila by promoting the formation of stress granules
Abstract
The kinase TOR is found in two complexes, TORC1, which is involved in growth control, and TORC2, whose roles are less well defined. Here, we asked whether TORC2 has a role in sustaining cellular stress. We show that TORC2 inhibition in Drosophila melanogaster leads to a reduced tolerance to heat stress, whereas sensitivity to other stresses is not affected. Accordingly, we show that upon heat stress, both in the animal and Drosophila cultured S2 cells, TORC2 is activated and is required for maintaining the level of its known target, Akt1 (also known as PKB). We show that the phosphorylation of the stress-activated protein kinases is not modulated by TORC2 nor is the heat-induced upregulation of heat-shock proteins. Instead, we show, both in vivo and in cultured cells, that TORC2 is required for the assembly of heat-induced cytoprotective ribonucleoprotein particles, the pro-survival stress granules. These granules are formed in response to protein translation inhibition imposed by heat stress that appears to be less efficient in the absence of TORC2 function. We propose that TORC2 mediates heat resistance in Drosophila by promoting the cell autonomous formation of stress granules.
Keywords: Akt; Drosophila S2 cells; Heat stress; Heat-shock protein; PKB; Rictor; SAPK; Sin1; Stress granules; TORC2; Translation.
© 2015. Published by The Company of Biologists Ltd.
Conflict of interest statement
The authors declare no competing or financial interests.
Figures
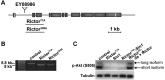
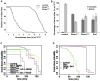
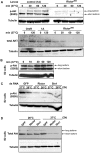
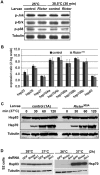
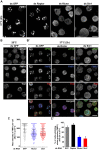
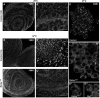
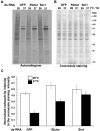
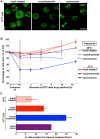
Similar articles
-
Tuberous sclerosis complex regulates Drosophila neuromuscular junction growth via the TORC2/Akt pathway.Hum Mol Genet. 2013 May 15;22(10):2010-23. doi: 10.1093/hmg/ddt053. Epub 2013 Feb 7. Hum Mol Genet. 2013. PMID: 23393158
-
Target of Rapamycin Complex 2 regulates cell growth via Myc in Drosophila.Sci Rep. 2015 May 22;5:10339. doi: 10.1038/srep10339. Sci Rep. 2015. PMID: 25999153 Free PMC article.
-
LST8 regulates cell growth via target-of-rapamycin complex 2 (TORC2).Mol Cell Biol. 2012 Jun;32(12):2203-13. doi: 10.1128/MCB.06474-11. Epub 2012 Apr 9. Mol Cell Biol. 2012. PMID: 22493059 Free PMC article.
-
Evolutionarily conserved regulation of TOR signalling.J Biochem. 2013 Jul;154(1):1-10. doi: 10.1093/jb/mvt047. Epub 2013 May 21. J Biochem. 2013. PMID: 23698095 Review.
-
Who does TORC2 talk to?Biochem J. 2018 May 24;475(10):1721-1738. doi: 10.1042/BCJ20180130. Biochem J. 2018. PMID: 29794170 Review.
Cited by
-
Role of gut commensal bacteria in juvenile developmental growth of the host: insights from Drosophila studies.Anim Cells Syst (Seoul). 2023 Nov 15;27(1):329-339. doi: 10.1080/19768354.2023.2282726. eCollection 2023. Anim Cells Syst (Seoul). 2023. PMID: 38023592 Free PMC article. Review.
-
Canonical nucleators are dispensable for stress granule assembly in Drosophila intestinal progenitors.J Cell Sci. 2020 May 18;133(10):jcs243451. doi: 10.1242/jcs.243451. J Cell Sci. 2020. PMID: 32265270 Free PMC article.
-
Heat tolerance in Drosophila melanogaster is influenced by oxygen conditions and mutations in cell size control pathways.Philos Trans R Soc Lond B Biol Sci. 2024 Feb 26;379(1896):20220490. doi: 10.1098/rstb.2022.0490. Epub 2024 Jan 8. Philos Trans R Soc Lond B Biol Sci. 2024. PMID: 38186282
-
Stress granules inhibit fatty acid oxidation by modulating mitochondrial permeability.Cell Rep. 2021 Jun 15;35(11):109237. doi: 10.1016/j.celrep.2021.109237. Cell Rep. 2021. PMID: 34133922 Free PMC article.
-
Mitochondrial ROS signalling requires uninterrupted electron flow and is lost during ageing in flies.Geroscience. 2022 Aug;44(4):1961-1974. doi: 10.1007/s11357-022-00555-x. Epub 2022 Mar 30. Geroscience. 2022. PMID: 35355221 Free PMC article.
References
Publication types
MeSH terms
Substances
LinkOut - more resources
Full Text Sources
Other Literature Sources
Molecular Biology Databases
Miscellaneous