Therapeutic effects of glatiramer acetate and grafted CD115⁺ monocytes in a mouse model of Alzheimer's disease
- PMID: 26049087
- PMCID: PMC4840949
- DOI: 10.1093/brain/awv150
Therapeutic effects of glatiramer acetate and grafted CD115⁺ monocytes in a mouse model of Alzheimer's disease
Abstract
Weekly glatiramer acetate immunization of transgenic mice modelling Alzheimer's disease resulted in retained cognition (Morris water maze test), decreased amyloid-β plaque burden, and regulation of local inflammation through a mechanism involving enhanced recruitment of monocytes. Ablation of bone marrow-derived myeloid cells exacerbated plaque pathology, whereas weekly administration of glatiramer acetate enhanced cerebral recruitment of innate immune cells, which dampened the pathology. Here, we assessed the therapeutic potential of grafted CD115(+) monocytes, injected once monthly into the peripheral blood of transgenic APPSWE/PS1ΔE9 Alzheimer's disease mouse models, with and without weekly immunization of glatiramer acetate, as compared to glatiramer acetate alone. All immune-modulation treatment groups were compared with age-matched phosphate-buffered saline-injected control transgenic and untreated non-transgenic mouse groups. Two independent cohorts of mice were assessed for behavioural performance (6-8 mice/group); treatments started in 10-month-old symptomatic mice and spanned a total of 2 months. For all three treatments, our data suggest a substantial decrease in cognitive deficit as assessed by the Barnes maze test (P < 0.0001-0.001). Improved cognitive function was associated with synaptic preservation and reduction in cerebral amyloid-β protein levels and astrogliosis (P < 0.001 and P < 0.0001), with no apparent additive effects for the combined treatment. The peripherally grafted, green fluorescent protein-labelled and endogenous monocytes, homed to cerebral amyloid plaques and directly engulfed amyloid-β; their recruitment was further enhanced by glatiramer acetate. In glatiramer acetate-immunized mice and, moreover, in the combined treatment group, monocyte recruitment to the brain was coupled with greater elevation of the regulatory cytokine IL10 surrounding amyloid-β plaques. All treated transgenic mice had increased cerebral levels of MMP9 protein (P < 0.05), an enzyme capable of degrading amyloid-β, which was highly expressed by the infiltrating monocytes. In vitro studies using primary cultures of bone marrow monocyte-derived macrophages, demonstrated that glatiramer acetate enhanced the ability of macrophages to phagocytose preformed fibrillar amyloid-β1-42 (P < 0.0001). These glatiramer acetate-treated macrophages exhibited increased expression of the scavenger receptors CD36 and SCARA1 (encoded by MSR1), which can facilitate amyloid-β phagocytosis, and the amyloid-β-degrading enzyme MMP9 (P < 0.0001-0.001). Overall, our studies indicate that increased cerebral infiltration of monocytes, either by enrichment of their levels in the circulation or by weekly immunization with glatiramer acetate, resulted in substantial attenuation of disease progression in murine Alzheimer's models by mechanisms that involved enhanced cellular uptake and enzymatic degradation of toxic amyloid-β as well as regulation of brain inflammation.
Keywords: Alzheimer’s disease; behavioural neurology; dementia; neuroinflammation; neuroprotection.
© The Author (2015). Published by Oxford University Press on behalf of the Guarantors of Brain. All rights reserved. For Permissions, please email: journals.permissions@oup.com.
Figures
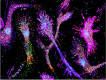
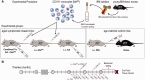
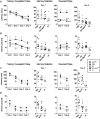
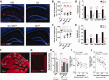
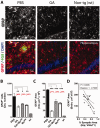
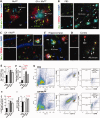
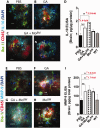
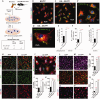
Similar articles
-
Peripherally derived angiotensin converting enzyme-enhanced macrophages alleviate Alzheimer-related disease.Brain. 2020 Jan 1;143(1):336-358. doi: 10.1093/brain/awz364. Brain. 2020. PMID: 31794021 Free PMC article.
-
A novel role for osteopontin in macrophage-mediated amyloid-β clearance in Alzheimer's models.Brain Behav Immun. 2018 Jan;67:163-180. doi: 10.1016/j.bbi.2017.08.019. Epub 2017 Aug 30. Brain Behav Immun. 2018. PMID: 28860067 Free PMC article.
-
Myeloid differentiation factor 88-deficient bone marrow cells improve Alzheimer's disease-related symptoms and pathology.Brain. 2011 Jan;134(Pt 1):278-92. doi: 10.1093/brain/awq325. Epub 2010 Nov 28. Brain. 2011. PMID: 21115468
-
Clearance of cerebral Aβ in Alzheimer's disease: reassessing the role of microglia and monocytes.Cell Mol Life Sci. 2017 Jun;74(12):2167-2201. doi: 10.1007/s00018-017-2463-7. Epub 2017 Feb 14. Cell Mol Life Sci. 2017. PMID: 28197669 Free PMC article. Review.
-
The effects of enhancing angiotensin converting enzyme in myelomonocytes on ameliorating Alzheimer's-related disease and preserving cognition.Front Physiol. 2023 Jun 23;14:1179315. doi: 10.3389/fphys.2023.1179315. eCollection 2023. Front Physiol. 2023. PMID: 37427403 Free PMC article. Review.
Cited by
-
Contextualizing the Role of Osteopontin in the Inflammatory Responses of Alzheimer's Disease.Biomedicines. 2023 Dec 6;11(12):3232. doi: 10.3390/biomedicines11123232. Biomedicines. 2023. PMID: 38137453 Free PMC article. Review.
-
N-acetylneuraminic acid links immune exhaustion and accelerated memory deficit in diet-induced obese Alzheimer's disease mouse model.Nat Commun. 2023 Mar 9;14(1):1293. doi: 10.1038/s41467-023-36759-8. Nat Commun. 2023. PMID: 36894557 Free PMC article.
-
The effects of high plasma levels of Aβ1-42 on mononuclear macrophage in mouse models of Alzheimer's disease.Immun Ageing. 2023 Jul 31;20(1):39. doi: 10.1186/s12979-023-00366-4. Immun Ageing. 2023. PMID: 37525137 Free PMC article.
-
Advances in Amyloid-β Clearance in the Brain and Periphery: Implications for Neurodegenerative Diseases.Exp Neurobiol. 2023 Aug 31;32(4):216-246. doi: 10.5607/en23014. Exp Neurobiol. 2023. PMID: 37749925 Free PMC article. Review.
-
Immunomodulation induced by central nervous system-related peptides as a therapeutic strategy for neurodegenerative disorders.Pharmacol Res Perspect. 2021 Oct;9(5):e00795. doi: 10.1002/prp2.795. Pharmacol Res Perspect. 2021. PMID: 34609083 Free PMC article. Review.
References
-
- Aharoni R, Sonego H, Brenner O, Eilam R, Arnon R. The therapeutic effect of glatiramer acetate in a murine model of inflammatory bowel disease is mediated by anti-inflammatory T-cells. Immunol Lett 2007; 112: 110–9. - PubMed
-
- Akiyama H, Arai T, Kondo H, Tanno E, Haga C, Ikeda K. Cell mediators of inflammation in the Alzheimer disease brain. Alzheimer Dis Assoc Disord 2000a; 14 Suppl 1: S47–53. - PubMed
-
- Bakalash S, Pham M, Koronyo Y, Salumbides BC, Kramerov A, Seidenberg H, et al. Egr1 expression is induced following glatiramer acetate immunotherapy in rodent models of glaucoma and Alzheimer's disease. Invest Ophthalmol Vis Sci 2011; 52: 9033–46. - PubMed
Publication types
MeSH terms
Substances
Grants and funding
LinkOut - more resources
Full Text Sources
Other Literature Sources
Medical
Miscellaneous