A modular open platform for systematic functional studies under physiological conditions
- PMID: 26007658
- PMCID: PMC4787826
- DOI: 10.1093/nar/gkv550
A modular open platform for systematic functional studies under physiological conditions
Abstract
Any profound comprehension of gene function requires detailed information about the subcellular localization, molecular interactions and spatio-temporal dynamics of gene products. We developed a multifunctional integrase (MIN) tag for rapid and versatile genome engineering that serves not only as a genetic entry site for the Bxb1 integrase but also as a novel epitope tag for standardized detection and precipitation. For the systematic study of epigenetic factors, including Dnmt1, Dnmt3a, Dnmt3b, Tet1, Tet2, Tet3 and Uhrf1, we generated MIN-tagged embryonic stem cell lines and created a toolbox of prefabricated modules that can be integrated via Bxb1-mediated recombination. We used these functional modules to study protein interactions and their spatio-temporal dynamics as well as gene expression and specific mutations during cellular differentiation and in response to external stimuli. Our genome engineering strategy provides a versatile open platform for efficient generation of multiple isogenic cell lines to study gene function under physiological conditions.
© The Author(s) 2015. Published by Oxford University Press on behalf of Nucleic Acids Research.
Figures
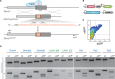
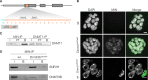
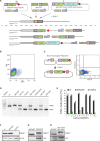
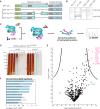
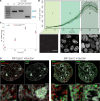
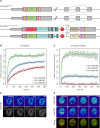
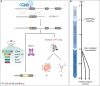
Similar articles
-
Comparison of Integrases Identifies Bxb1-GA Mutant as the Most Efficient Site-Specific Integrase System in Mammalian Cells.ACS Synth Biol. 2019 Jan 18;8(1):16-24. doi: 10.1021/acssynbio.8b00089. Epub 2019 Jan 9. ACS Synth Biol. 2019. PMID: 30609349
-
Creation of engineered human embryonic stem cell lines using phiC31 integrase.Stem Cells. 2008 Jan;26(1):119-26. doi: 10.1634/stemcells.2007-0283. Epub 2007 Oct 25. Stem Cells. 2008. PMID: 17962703
-
An open-source system for in planta gene stacking by Bxb1 and Cre recombinases.Mol Plant. 2014 Dec;7(12):1756-65. doi: 10.1093/mp/ssu107. Epub 2014 Oct 3. Mol Plant. 2014. PMID: 25281665
-
Generation of eGFP and Cre knockin rats by CRISPR/Cas9.FEBS J. 2014 Sep;281(17):3779-90. doi: 10.1111/febs.12935. Epub 2014 Aug 13. FEBS J. 2014. PMID: 25039742
-
Recombinase-mediated cassette exchange (RMCE) - a rapidly-expanding toolbox for targeted genomic modifications.Gene. 2013 Feb 15;515(1):1-27. doi: 10.1016/j.gene.2012.11.016. Epub 2012 Nov 29. Gene. 2013. PMID: 23201421 Review.
Cited by
-
METTL6 is a tRNA m3C methyltransferase that regulates pluripotency and tumor cell growth.Sci Adv. 2020 Aug 26;6(35):eaaz4551. doi: 10.1126/sciadv.aaz4551. eCollection 2020 Aug. Sci Adv. 2020. PMID: 32923617 Free PMC article.
-
Identification of permissive amber suppression sites for efficient non-canonical amino acid incorporation in mammalian cells.Nucleic Acids Res. 2021 Jun 21;49(11):e62. doi: 10.1093/nar/gkab132. Nucleic Acids Res. 2021. PMID: 33684219 Free PMC article.
-
The termination of UHRF1-dependent PAF15 ubiquitin signaling is regulated by USP7 and ATAD5.Elife. 2023 Feb 3;12:e79013. doi: 10.7554/eLife.79013. Elife. 2023. PMID: 36734974 Free PMC article.
-
HP1β carries an acidic linker domain and requires H3K9me3 for phase separation.Nucleus. 2021 Dec;12(1):44-57. doi: 10.1080/19491034.2021.1889858. Nucleus. 2021. PMID: 33660589 Free PMC article.
-
Multivalent binding of PWWP2A to H2A.Z regulates mitosis and neural crest differentiation.EMBO J. 2017 Aug 1;36(15):2263-2279. doi: 10.15252/embj.201695757. Epub 2017 Jun 23. EMBO J. 2017. PMID: 28645917 Free PMC article.
References
-
- Kuscu C., Arslan S., Singh R., Thorpe J., Adli M. Genome-wide analysis reveals characteristics of off-target sites bound by the Cas9 endonuclease. Nat. Biotechnol. 2014;32:677–683. - PubMed
Publication types
MeSH terms
Substances
LinkOut - more resources
Full Text Sources
Other Literature Sources
Research Materials