Towards a Unified Theory of Calmodulin Regulation (Calmodulation) of Voltage-Gated Calcium and Sodium Channels
- PMID: 25966688
- PMCID: PMC4960983
- DOI: 10.2174/1874467208666150507110359
Towards a Unified Theory of Calmodulin Regulation (Calmodulation) of Voltage-Gated Calcium and Sodium Channels
Abstract
Voltage-gated Na and Ca(2+) channels represent two major ion channel families that enable myriad biological functions including the generation of action potentials and the coupling of electrical and chemical signaling in cells. Calmodulin regulation (calmodulation) of these ion channels comprises a vital feedback mechanism with distinct physiological implications. Though long-sought, a shared understanding of the channel families remained elusive for two decades as the functional manifestations and the structural underpinnings of this modulation often appeared to diverge. Here, we review recent advancements in the understanding of calmodulation of Ca(2+) and Na channels that suggest a remarkable similarity in their regulatory scheme. This interrelation between the two channel families now paves the way towards a unified mechanistic framework to understand vital calmodulin-dependent feedback and offers shared principles to approach related channelopathic diseases. An exciting era of synergistic study now looms.
Figures
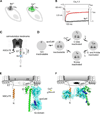
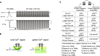
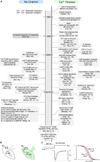
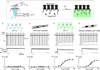
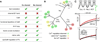
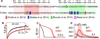
Similar articles
-
Calmodulin regulation (calmodulation) of voltage-gated calcium channels.J Gen Physiol. 2014 Jun;143(6):679-92. doi: 10.1085/jgp.201311153. J Gen Physiol. 2014. PMID: 24863929 Free PMC article. Review.
-
Conservation of Ca2+/calmodulin regulation across Na and Ca2+ channels.Cell. 2014 Jun 19;157(7):1657-70. doi: 10.1016/j.cell.2014.04.035. Cell. 2014. PMID: 24949975 Free PMC article.
-
Bilobal architecture is a requirement for calmodulin signaling to CaV1.3 channels.Proc Natl Acad Sci U S A. 2018 Mar 27;115(13):E3026-E3035. doi: 10.1073/pnas.1716381115. Epub 2018 Mar 12. Proc Natl Acad Sci U S A. 2018. PMID: 29531055 Free PMC article.
-
Seeing the forest through the trees: towards a unified view on physiological calcium regulation of voltage-gated sodium channels.Biophys J. 2012 Dec 5;103(11):2243-51. doi: 10.1016/j.bpj.2012.10.020. Biophys J. 2012. PMID: 23283222 Free PMC article. Review.
-
Current view on regulation of voltage-gated sodium channels by calcium and auxiliary proteins.Protein Sci. 2016 Sep;25(9):1573-84. doi: 10.1002/pro.2960. Epub 2016 Jun 13. Protein Sci. 2016. PMID: 27262167 Free PMC article. Review.
Cited by
-
Calmodulin binds to the N-terminal domain of the cardiac sodium channel Nav1.5.Channels (Austin). 2020 Dec;14(1):268-286. doi: 10.1080/19336950.2020.1805999. Channels (Austin). 2020. PMID: 32815768 Free PMC article.
-
Voltage-Gated Calcium Channels: Key Players in Sensory Coding in the Retina and the Inner Ear.Physiol Rev. 2018 Oct 1;98(4):2063-2096. doi: 10.1152/physrev.00030.2017. Physiol Rev. 2018. PMID: 30067155 Free PMC article. Review.
-
Calmodulinopathy: A Novel, Life-Threatening Clinical Entity Affecting the Young.Front Cardiovasc Med. 2018 Dec 6;5:175. doi: 10.3389/fcvm.2018.00175. eCollection 2018. Front Cardiovasc Med. 2018. PMID: 30574507 Free PMC article. Review.
-
Calmodulin mutations and life-threatening cardiac arrhythmias: insights from the International Calmodulinopathy Registry.Eur Heart J. 2019 Sep 14;40(35):2964-2975. doi: 10.1093/eurheartj/ehz311. Eur Heart J. 2019. PMID: 31170290 Free PMC article.
-
Calcium channel gating.Pflugers Arch. 2018 Sep;470(9):1291-1309. doi: 10.1007/s00424-018-2163-7. Epub 2018 Jun 27. Pflugers Arch. 2018. PMID: 29951751 Free PMC article. Review.
References
-
- Saimi Y, Kung C. Calmodulin as an ion channel subunit. Annu. Rev. Physiol. 2002;64:289–311. - PubMed
-
- Kink JA, Maley ME, Preston RR, Ling KY, Wallen-Friedman MA, Saimi Y, Kung C. Mutations in paramecium calmodulin indicate functional differences between the C-terminal and N-terminal lobes in vivo. Cell. 1990;62:165–174. - PubMed
-
- Saimi Y, Kung C. Ion channel regulation by calmodulin binding. FEBS Lett. 1994;350:155–158. - PubMed
-
- Adelman JP, Maylie J, Sah P. Small-conductance Ca2+-activated K+ channels: form and function. Annu. Rev. Physiol. 2012;74:245–269. - PubMed
Publication types
MeSH terms
Substances
Grants and funding
LinkOut - more resources
Full Text Sources
Miscellaneous