An acidic microenvironment sets the humoral pattern recognition molecule PTX3 in a tissue repair mode
- PMID: 25964372
- PMCID: PMC4451130
- DOI: 10.1084/jem.20141268
An acidic microenvironment sets the humoral pattern recognition molecule PTX3 in a tissue repair mode
Abstract
Pentraxin 3 (PTX3) is a fluid-phase pattern recognition molecule and a key component of the humoral arm of innate immunity. In four different models of tissue damage in mice, PTX3 deficiency was associated with increased fibrin deposition and persistence, and thicker clots, followed by increased collagen deposition, when compared with controls. Ptx3-deficient macrophages showed defective pericellular fibrinolysis in vitro. PTX3-bound fibrinogen/fibrin and plasminogen at acidic pH and increased plasmin-mediated fibrinolysis. The second exon-encoded N-terminal domain of PTX3 recapitulated the activity of the intact molecule. Thus, a prototypic component of humoral innate immunity, PTX3, plays a nonredundant role in the orchestration of tissue repair and remodeling. Tissue acidification resulting from metabolic adaptation during tissue repair sets PTX3 in a tissue remodeling and repair mode, suggesting that matrix and microbial recognition are common, ancestral features of the humoral arm of innate immunity.
© 2015 Doni et al.
Figures
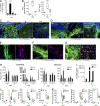
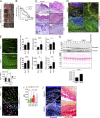
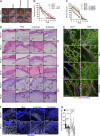
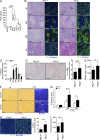
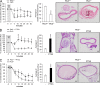
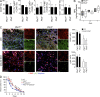
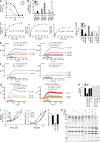
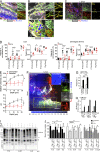
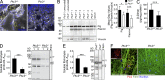
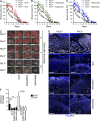
Comment in
-
Pentraxin 3 innately preps damaged tissue for wound healing.J Exp Med. 2015 Jun 1;212(6):829. doi: 10.1084/jem.2126insight2. J Exp Med. 2015. PMID: 26034115 Free PMC article. No abstract available.
Similar articles
-
Humoral innate immunity at the crossroad between microbe and matrix recognition: The role of PTX3 in tissue damage.Semin Cell Dev Biol. 2017 Jan;61:31-40. doi: 10.1016/j.semcdb.2016.07.026. Epub 2016 Jul 29. Semin Cell Dev Biol. 2017. PMID: 27476448 Free PMC article. Review.
-
PTX3 Regulation of Inflammation, Hemostatic Response, Tissue Repair, and Resolution of Fibrosis Favors a Role in Limiting Idiopathic Pulmonary Fibrosis.Front Immunol. 2021 Jun 21;12:676702. doi: 10.3389/fimmu.2021.676702. eCollection 2021. Front Immunol. 2021. PMID: 34276664 Free PMC article. Review.
-
Mesenchymal Stromal Cell-Derived PTX3 Promotes Wound Healing via Fibrin Remodeling.J Invest Dermatol. 2016 Jan;136(1):293-300. doi: 10.1038/JID.2015.346. J Invest Dermatol. 2016. PMID: 26763449
-
PTX3, a Humoral Pattern Recognition Molecule, in Innate Immunity, Tissue Repair, and Cancer.Physiol Rev. 2018 Apr 1;98(2):623-639. doi: 10.1152/physrev.00016.2017. Physiol Rev. 2018. PMID: 29412047 Free PMC article.
-
The long pentraxin PTX3 at the crossroads between innate immunity and tissue remodelling.Tissue Antigens. 2011 Apr;77(4):271-82. doi: 10.1111/j.1399-0039.2011.01645.x. Tissue Antigens. 2011. PMID: 21388349
Cited by
-
Editorial: Interactions of Pentraxins and Complement in Infection, Inflammation, and Cancer.Front Immunol. 2022 Feb 17;13:861359. doi: 10.3389/fimmu.2022.861359. eCollection 2022. Front Immunol. 2022. PMID: 35251053 Free PMC article. No abstract available.
-
The Long Pentraxin PTX3 as a Humoral Innate Immunity Functional Player and Biomarker of Infections and Sepsis.Front Immunol. 2019 Apr 12;10:794. doi: 10.3389/fimmu.2019.00794. eCollection 2019. Front Immunol. 2019. PMID: 31031772 Free PMC article. Review.
-
Evaluating the Expression of Candidate Homeobox Genes and Their Role in Local-Site Inflammation in Mucosal Tissue Obtained from Children with Non-Syndromic Cleft Lip and Palate.J Pers Med. 2021 Nov 2;11(11):1135. doi: 10.3390/jpm11111135. J Pers Med. 2021. PMID: 34834487 Free PMC article.
-
TNF up-regulates Pentraxin3 expression in human airway smooth muscle cells via JNK and ERK1/2 MAPK pathways.Allergy Asthma Clin Immunol. 2015 Dec 7;11:37. doi: 10.1186/s13223-015-0104-y. eCollection 2015. Allergy Asthma Clin Immunol. 2015. PMID: 26644796 Free PMC article.
-
Fluid phase recognition molecules in neutrophil-dependent immune responses.Semin Immunol. 2016 Apr;28(2):109-18. doi: 10.1016/j.smim.2016.03.005. Epub 2016 Mar 25. Semin Immunol. 2016. PMID: 27021644 Free PMC article. Review.
References
-
- Barbieri S.S., Amadio P., Gianellini S., Tarantino E., Zacchi E., Veglia F., Howe L.R., Weksler B.B., Mussoni L., and Tremoli E.. 2012. Cyclooxygenase-2-derived prostacyclin regulates arterial thrombus formation by suppressing tissue factor in a sirtuin-1-dependent-manner. Circulation. 126:1373–1384. 10.1161/CIRCULATIONAHA.112.097295 - DOI - PubMed
-
- Bottazzi B., Vouret-Craviari V., Bastone A., De Gioia L., Matteucci C., Peri G., Spreafico F., Pausa M., D’Ettorre C., Gianazza E., et al. . 1997. Multimer formation and ligand recognition by the long pentraxin PTX3. Similarities and differences with the short pentraxins C-reactive protein and serum amyloid P component. J. Biol. Chem. 272:32817–32823. 10.1074/jbc.272.52.32817 - DOI - PubMed
Publication types
MeSH terms
Substances
LinkOut - more resources
Full Text Sources
Other Literature Sources
Molecular Biology Databases
Miscellaneous