Mechanical heterogeneity favors fragmentation of strained actin filaments
- PMID: 25954884
- PMCID: PMC4423049
- DOI: 10.1016/j.bpj.2015.03.058
Mechanical heterogeneity favors fragmentation of strained actin filaments
Abstract
We present a general model of actin filament deformation and fragmentation in response to compressive forces. The elastic free energy density along filaments is determined by their shape and mechanical properties, which were modeled in terms of bending, twisting, and twist-bend coupling elasticities. The elastic energy stored in filament deformation (i.e., strain) tilts the fragmentation-annealing reaction free-energy profile to favor fragmentation. The energy gradient introduces a local shear force that accelerates filament intersubunit bond rupture. The severing protein, cofilin, renders filaments more compliant in bending and twisting. As a result, filaments that are partially decorated with cofilin are mechanically heterogeneous (i.e., nonuniform) and display asymmetric shape deformations and energy profiles distinct from mechanically homogenous (i.e., uniform), bare actin, or saturated cofilactin filaments. The local buckling strain depends on the relative size of the compliant segment as well as the bending and twisting rigidities of flanking regions. Filaments with a single bare/cofilin-decorated boundary localize energy and force adjacent to the boundary, within the compliant cofilactin segment. Filaments with small cofilin clusters were predicted to fragment within the compliant cofilactin rather than at boundaries. Neglecting contributions from twist-bend coupling elasticity underestimates the energy density and gradients along filaments, and thus the net effects of filament strain to fragmentation. Spatial confinement causes compliant cofilactin segments and filaments to adopt higher deformation modes and store more elastic energy, thereby promoting fragmentation. The theory and simulations presented here establish a quantitative relationship between actin filament fragmentation thermodynamics and elasticity, and reveal how local discontinuities in filament mechanical properties introduced by regulatory proteins can modulate both the severing efficiency and location along filaments. The emergent behavior of mechanically heterogeneous filaments, particularly under confinement, emphasizes that severing in cells is likely to be influenced by multiple physical and chemical factors.
Copyright © 2015 Biophysical Society. Published by Elsevier Inc. All rights reserved.
Figures
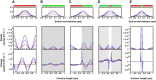
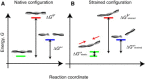
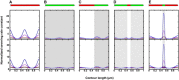
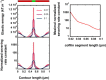
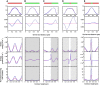
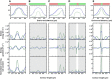
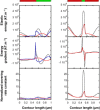
Comment in
-
Forcing filament fragmentation with cofilin.Biophys J. 2015 May 5;108(9):2094-5. doi: 10.1016/j.bpj.2015.04.002. Biophys J. 2015. PMID: 25954866 Free PMC article. No abstract available.
Similar articles
-
Actin Filament Strain Promotes Severing and Cofilin Dissociation.Biophys J. 2017 Jun 20;112(12):2624-2633. doi: 10.1016/j.bpj.2017.05.016. Biophys J. 2017. PMID: 28636918 Free PMC article.
-
Cofilin-linked changes in actin filament flexibility promote severing.Biophys J. 2011 Jul 6;101(1):151-9. doi: 10.1016/j.bpj.2011.05.049. Biophys J. 2011. PMID: 21723825 Free PMC article.
-
Plastic Deformation and Fragmentation of Strained Actin Filaments.Biophys J. 2019 Aug 6;117(3):453-463. doi: 10.1016/j.bpj.2019.06.018. Epub 2019 Jun 25. Biophys J. 2019. PMID: 31301801 Free PMC article.
-
Biophysics of actin filament severing by cofilin.FEBS Lett. 2013 Apr 17;587(8):1215-9. doi: 10.1016/j.febslet.2013.01.062. Epub 2013 Feb 5. FEBS Lett. 2013. PMID: 23395798 Free PMC article. Review.
-
Regulation of actin filament dynamics by actin depolymerizing factor/cofilin and actin-interacting protein 1: new blades for twisted filaments.Biochemistry. 2003 Nov 25;42(46):13363-70. doi: 10.1021/bi034600x. Biochemistry. 2003. PMID: 14621980 Review.
Cited by
-
Forcing filament fragmentation with cofilin.Biophys J. 2015 May 5;108(9):2094-5. doi: 10.1016/j.bpj.2015.04.002. Biophys J. 2015. PMID: 25954866 Free PMC article. No abstract available.
-
Computational modeling of single-cell mechanics and cytoskeletal mechanobiology.Wiley Interdiscip Rev Syst Biol Med. 2018 Mar;10(2):e1407. doi: 10.1002/wsbm.1407. Epub 2017 Nov 30. Wiley Interdiscip Rev Syst Biol Med. 2018. PMID: 29195023 Free PMC article. Review.
-
Thermal fracture kinetics of heterogeneous semiflexible polymers.Soft Matter. 2020 Feb 26;16(8):2017-2024. doi: 10.1039/c9sm01637f. Soft Matter. 2020. PMID: 31996875 Free PMC article.
-
Catastrophic actin filament bursting by cofilin, Aip1, and coronin.J Biol Chem. 2020 Sep 18;295(38):13299-13313. doi: 10.1074/jbc.RA120.015018. Epub 2020 Jul 28. J Biol Chem. 2020. PMID: 32723865 Free PMC article.
-
Phosphomimetic S3D cofilin binds but only weakly severs actin filaments.J Biol Chem. 2017 Dec 1;292(48):19565-19579. doi: 10.1074/jbc.M117.808378. Epub 2017 Sep 22. J Biol Chem. 2017. PMID: 28939776 Free PMC article.
References
Publication types
MeSH terms
Substances
Grants and funding
LinkOut - more resources
Full Text Sources
Other Literature Sources
Research Materials