Intracellular FGF14 (iFGF14) Is Required for Spontaneous and Evoked Firing in Cerebellar Purkinje Neurons and for Motor Coordination and Balance
- PMID: 25926453
- PMCID: PMC4412895
- DOI: 10.1523/JNEUROSCI.2663-14.2015
Intracellular FGF14 (iFGF14) Is Required for Spontaneous and Evoked Firing in Cerebellar Purkinje Neurons and for Motor Coordination and Balance
Abstract
Mutations in FGF14, which encodes intracellular fibroblast growth factor 14 (iFGF14), have been linked to spinocerebellar ataxia (SCA27). In addition, mice lacking Fgf14 (Fgf14(-/-)) exhibit an ataxia phenotype resembling SCA27, accompanied by marked changes in the excitability of cerebellar granule and Purkinje neurons. It is not known, however, whether these phenotypes result from defects in neuronal development or if they reflect a physiological requirement for iFGF14 in the adult cerebellum. Here, we demonstrate that the acute and selective Fgf14-targeted short hairpin RNA (shRNA)-mediated in vivo "knock-down" of iFGF14 in adult Purkinje neurons attenuates spontaneous and evoked action potential firing without measurably affecting the expression or localization of voltage-gated Na(+) (Nav) channels at Purkinje neuron axon initial segments. The selective shRNA-mediated in vivo "knock-down" of iFGF14 in adult Purkinje neurons also impairs motor coordination and balance. Repetitive firing can be restored in Fgf14-targeted shRNA-expressing Purkinje neurons, as well as in Fgf14(-/-) Purkinje neurons, by prior membrane hyperpolarization, suggesting that the iFGF14-mediated regulation of the excitability of mature Purkinje neurons depends on membrane potential. Further experiments revealed that the loss of iFGF14 results in a marked hyperpolarizing shift in the voltage dependence of steady-state inactivation of the Nav currents in adult Purkinje neurons. We also show here that expressing iFGF14 selectively in adult Fgf14(-/-) Purkinje neurons rescues spontaneous firing and improves motor performance. Together, these results demonstrate that iFGF14 is required for spontaneous and evoked action potential firing in adult Purkinje neurons, thereby controlling the output of these cells and the regulation of motor coordination and balance.
Keywords: FGF14; channel inactivation; fibroblast growth factor homologous factor 4 (FHF4); intrinsic excitability; spinocerebellar ataxia 27; voltage-gated sodium (Nav) channels.
Copyright © 2015 the authors 0270-6474/15/356752-18$15.00/0.
Figures
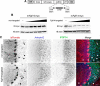
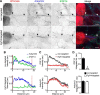
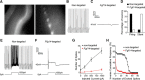
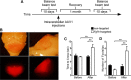
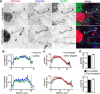
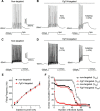
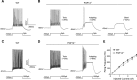
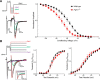
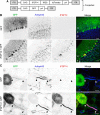
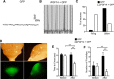
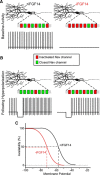
Similar articles
-
FGF14 regulates the intrinsic excitability of cerebellar Purkinje neurons.Neurobiol Dis. 2009 Jan;33(1):81-8. doi: 10.1016/j.nbd.2008.09.019. Epub 2008 Oct 1. Neurobiol Dis. 2009. PMID: 18930825 Free PMC article.
-
In Vivo Expression of an SCA27A-linked FGF14 Mutation Results in Haploinsufficiency and Impaired Firing of Cerebellar Purkinje Neurons.bioRxiv [Preprint]. 2024 Oct 25:2024.10.25.620253. doi: 10.1101/2024.10.25.620253. bioRxiv. 2024. PMID: 39484407 Free PMC article. Preprint.
-
Loss of Intracellular Fibroblast Growth Factor 14 (iFGF14) Increases the Excitability of Mature Hippocampal and Cortical Pyramidal Neurons.bioRxiv [Preprint]. 2024 May 4:2024.05.04.592532. doi: 10.1101/2024.05.04.592532. bioRxiv. 2024. PMID: 38746081 Free PMC article. Preprint.
-
Voltage-gated sodium currents in cerebellar Purkinje neurons: functional and molecular diversity.Cell Mol Life Sci. 2018 Oct;75(19):3495-3505. doi: 10.1007/s00018-018-2868-y. Epub 2018 Jul 7. Cell Mol Life Sci. 2018. PMID: 29982847 Free PMC article. Review.
-
Intracellular Fibroblast Growth Factor 14: Emerging Risk Factor for Brain Disorders.Front Cell Neurosci. 2017 Apr 19;11:103. doi: 10.3389/fncel.2017.00103. eCollection 2017. Front Cell Neurosci. 2017. PMID: 28469558 Free PMC article. Review.
Cited by
-
Temporal mapping of derived high-frequency gene variants supports the mosaic nature of the evolution of Homo sapiens.Sci Rep. 2022 Jun 15;12(1):9937. doi: 10.1038/s41598-022-13589-0. Sci Rep. 2022. PMID: 35705575 Free PMC article.
-
Fast inactivation of Nav current in rat adrenal chromaffin cells involves two independent inactivation pathways.J Gen Physiol. 2021 Apr 5;153(4):e202012784. doi: 10.1085/jgp.202012784. J Gen Physiol. 2021. PMID: 33647101 Free PMC article.
-
Episodic Ataxias: Faux or Real?Int J Mol Sci. 2020 Sep 5;21(18):6472. doi: 10.3390/ijms21186472. Int J Mol Sci. 2020. PMID: 32899446 Free PMC article. Review.
-
Changes in the Fluorescence Tracking of NaV1.6 Protein Expression in a BTBR T+Itpr3tf/J Autistic Mouse Model.Neural Plast. 2019 Dec 17;2019:4893103. doi: 10.1155/2019/4893103. eCollection 2019. Neural Plast. 2019. PMID: 31933626 Free PMC article.
-
A novel, ataxic mouse model of ataxia telangiectasia caused by a clinically relevant nonsense mutation.Elife. 2021 Nov 1;10:e64695. doi: 10.7554/eLife.64695. Elife. 2021. PMID: 34723800 Free PMC article.
References
-
- Buttermore ED, Piochon C, Wallace ML, Philpot BD, Hansel C, Bhat MA. Pinceau organization in the cerebellum requires distinct functions of neurofascin in Purkinje and basket neurons during postnatal development. J Neurosci. 2012;32:4724–4742. doi: 10.1523/JNEUROSCI.5602-11.2012. - DOI - PMC - PubMed
Publication types
MeSH terms
Substances
Grants and funding
LinkOut - more resources
Full Text Sources
Molecular Biology Databases