Bioengineering Thymus Organoids to Restore Thymic Function and Induce Donor-Specific Immune Tolerance to Allografts
- PMID: 25903472
- PMCID: PMC4817796
- DOI: 10.1038/mt.2015.77
Bioengineering Thymus Organoids to Restore Thymic Function and Induce Donor-Specific Immune Tolerance to Allografts
Abstract
One of the major obstacles in organ transplantation is to establish immune tolerance of allografts. Although immunosuppressive drugs can prevent graft rejection to a certain degree, their efficacies are limited, transient, and associated with severe side effects. Induction of thymic central tolerance to allografts remains challenging, largely because of the difficulty of maintaining donor thymic epithelial cells in vitro to allow successful bioengineering. Here, the authors show that three-dimensional scaffolds generated from decellularized mouse thymus can support thymic epithelial cell survival in culture and maintain their unique molecular properties. When transplanted into athymic nude mice, the bioengineered thymus organoids effectively promoted homing of lymphocyte progenitors and supported thymopoiesis. Nude mice transplanted with thymus organoids promptly rejected skin allografts and were able to mount antigen-specific humoral responses against ovalbumin on immunization. Notably, tolerance to skin allografts was achieved by transplanting thymus organoids constructed with either thymic epithelial cells coexpressing both syngeneic and allogenic major histocompatibility complexes, or mixtures of donor and recipient thymic epithelial cells. Our results demonstrate the technical feasibility of restoring thymic function with bioengineered thymus organoids and highlight the clinical implications of this thymus reconstruction technique in organ transplantation and regenerative medicine.
Figures
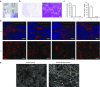
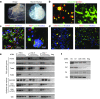
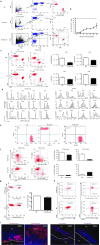
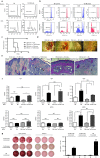
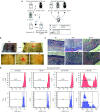
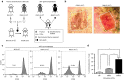
Similar articles
-
Construction of Thymus Organoids from Decellularized Thymus Scaffolds.Methods Mol Biol. 2019;1576:33-42. doi: 10.1007/7651_2016_9. Methods Mol Biol. 2019. PMID: 27730537 Free PMC article.
-
Native thymic extracellular matrix improves in vivo thymic organoid T cell output, and drives in vitro thymic epithelial cell differentiation.Biomaterials. 2017 Feb;118:1-15. doi: 10.1016/j.biomaterials.2016.11.054. Epub 2016 Nov 30. Biomaterials. 2017. PMID: 27940379
-
Thymic epithelium and the induction of transplantation tolerance in nude mice.Nature. 1985 Apr 4-10;314(6010):454-6. doi: 10.1038/314454a0. Nature. 1985. PMID: 3157063
-
Modulating Thymic Negative Selection with Bioengineered Thymus Organoids.In: Soboloff J, Kappes DJ, editors. Signaling Mechanisms Regulating T Cell Diversity and Function. Boca Raton (FL): CRC Press/Taylor & Francis; 2018. Chapter 4. In: Soboloff J, Kappes DJ, editors. Signaling Mechanisms Regulating T Cell Diversity and Function. Boca Raton (FL): CRC Press/Taylor & Francis; 2018. Chapter 4. PMID: 30427636 Free Books & Documents. Review.
-
[Culture and application of thymic organoids: a review].Sheng Wu Gong Cheng Xue Bao. 2021 Nov 25;37(11):3945-3960. doi: 10.13345/j.cjb.210071. Sheng Wu Gong Cheng Xue Bao. 2021. PMID: 34841797 Review. Chinese.
Cited by
-
The potential role of the thymus in immunotherapies for acute myeloid leukemia.Front Immunol. 2023 Feb 6;14:1102517. doi: 10.3389/fimmu.2023.1102517. eCollection 2023. Front Immunol. 2023. PMID: 36814919 Free PMC article. Review.
-
Mimicking tumor hypoxia and tumor-immune interactions employing three-dimensional in vitro models.J Exp Clin Cancer Res. 2020 May 1;39(1):75. doi: 10.1186/s13046-020-01583-1. J Exp Clin Cancer Res. 2020. PMID: 32357910 Free PMC article. Review.
-
Thymic function in the regulation of T cells, and molecular mechanisms underlying the modulation of cytokines and stress signaling (Review).Mol Med Rep. 2017 Nov;16(5):7175-7184. doi: 10.3892/mmr.2017.7525. Epub 2017 Sep 19. Mol Med Rep. 2017. PMID: 28944829 Free PMC article. Review.
-
Restoration of Thymus Function with Bioengineered Thymus Organoids.Curr Stem Cell Rep. 2016 Jun;2(2):128-139. doi: 10.1007/s40778-016-0040-x. Curr Stem Cell Rep. 2016. PMID: 27529056 Free PMC article.
-
Central tolerance promoted by cell chimerism.Proc Natl Acad Sci U S A. 2022 Dec 27;119(52):e2214989119. doi: 10.1073/pnas.2214989119. Epub 2022 Dec 19. Proc Natl Acad Sci U S A. 2022. PMID: 36534805 Free PMC article.
References
-
- Fink, PJ (2013). The biology of recent thymic emigrants. Annu Rev Immunol 31: 31–50. - PubMed
-
- Boehm, T (2008). Thymus development and function. Curr Opin Immunol 20: 178–184. - PubMed
-
- Heng, TS, Chidgey, AP and Boyd, RL (2010). Getting back at nature: understanding thymic development and overcoming its atrophy. Curr Opin Pharmacol 10: 425–433. - PubMed
-
- Fletcher, AL, Calder, A, Hince, MN, Boyd, RL and Chidgey, AP (2011). The contribution of thymic stromal abnormalities to autoimmune disease. Crit Rev Immunol 31: 171–187. - PubMed
-
- Takada, K, Ohigashi, I, Kasai, M, Nakase, H and Takahama, Y (2014). Development and function of cortical thymic epithelial cells. Curr Top Microbiol Immunol 373: 1–17. - PubMed
Publication types
MeSH terms
Grants and funding
LinkOut - more resources
Full Text Sources
Other Literature Sources