C-Nap1 mutation affects centriole cohesion and is associated with a Seckel-like syndrome in cattle
- PMID: 25902731
- PMCID: PMC4423223
- DOI: 10.1038/ncomms7894
C-Nap1 mutation affects centriole cohesion and is associated with a Seckel-like syndrome in cattle
Abstract
Caprine-like Generalized Hypoplasia Syndrome (SHGC) is an autosomal-recessive disorder in Montbéliarde cattle. Affected animals present a wide range of clinical features that include the following: delayed development with low birth weight, hind limb muscular hypoplasia, caprine-like thin head and partial coat depigmentation. Here we show that SHGC is caused by a truncating mutation in the CEP250 gene that encodes the centrosomal protein C-Nap1. This mutation results in centrosome splitting, which neither affects centriole ultrastructure and duplication in dividing cells nor centriole function in cilium assembly and mitotic spindle organization. Loss of C-Nap1-mediated centriole cohesion leads to an altered cell migration phenotype. This discovery extends the range of loci that constitute the spectrum of autosomal primary recessive microcephaly (MCPH) and Seckel-like syndromes.
Figures
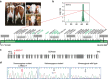
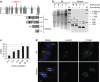
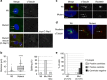
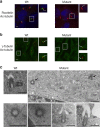
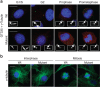
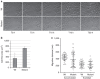
Similar articles
-
C-NAP1 and rootletin restrain DNA damage-induced centriole splitting and facilitate ciliogenesis.Cell Cycle. 2012 Oct 15;11(20):3769-78. doi: 10.4161/cc.21986. Cell Cycle. 2012. PMID: 23070519 Free PMC article.
-
Spindle positioning in human cells relies on proper centriole formation and on the microcephaly proteins CPAP and STIL.J Cell Sci. 2011 Nov 15;124(Pt 22):3884-93. doi: 10.1242/jcs.089888. Epub 2011 Nov 18. J Cell Sci. 2011. PMID: 22100914
-
Centlein mediates an interaction between C-Nap1 and Cep68 to maintain centrosome cohesion.J Cell Sci. 2014 Apr 15;127(Pt 8):1631-9. doi: 10.1242/jcs.139451. Epub 2014 Feb 19. J Cell Sci. 2014. PMID: 24554434
-
The centrosome duplication cycle in health and disease.FEBS Lett. 2014 Aug 1;588(15):2366-72. doi: 10.1016/j.febslet.2014.06.030. Epub 2014 Jun 18. FEBS Lett. 2014. PMID: 24951839 Review.
-
Comprehensive review on the molecular genetics of autosomal recessive primary microcephaly (MCPH).Genet Res (Camb). 2018 Aug 8;100:e7. doi: 10.1017/S0016672318000046. Genet Res (Camb). 2018. PMID: 30086807 Free PMC article. Review.
Cited by
-
The Centrosome and the Primary Cilium: The Yin and Yang of a Hybrid Organelle.Cells. 2019 Jul 10;8(7):701. doi: 10.3390/cells8070701. Cells. 2019. PMID: 31295970 Free PMC article. Review.
-
CROCCP2 acts as a human-specific modifier of cilia dynamics and mTOR signaling to promote expansion of cortical progenitors.Neuron. 2023 Jan 4;111(1):65-80.e6. doi: 10.1016/j.neuron.2022.10.018. Epub 2022 Nov 4. Neuron. 2023. PMID: 36334595 Free PMC article.
-
Centrosome linker protein C-Nap1 maintains stem cells in mouse testes.EMBO Rep. 2022 Jul 5;23(7):e53805. doi: 10.15252/embr.202153805. Epub 2022 May 23. EMBO Rep. 2022. PMID: 35599622 Free PMC article.
-
Mitotic Regulation by NEK Kinase Networks.Front Cell Dev Biol. 2017 Dec 1;5:102. doi: 10.3389/fcell.2017.00102. eCollection 2017. Front Cell Dev Biol. 2017. PMID: 29250521 Free PMC article. Review.
-
Bystanders or active players: the role of extra centrosomes as signaling hubs.Cancer Metastasis Rev. 2024 Nov 20;44(1):1. doi: 10.1007/s10555-024-10224-4. Cancer Metastasis Rev. 2024. PMID: 39570514 Free PMC article. Review.
References
Publication types
MeSH terms
Substances
Associated data
LinkOut - more resources
Full Text Sources
Other Literature Sources
Medical