Thiopurines induce oxidative stress in T-lymphocytes: a proteomic approach
- PMID: 25873760
- PMCID: PMC4385670
- DOI: 10.1155/2015/434825
Thiopurines induce oxidative stress in T-lymphocytes: a proteomic approach
Abstract
Thiopurines are extensively used immunosuppressants for the treatment of inflammatory bowel disease (IBD). The polymorphism of thiopurine S-methyltransferase (TPMT) influences thiopurine metabolism and therapy outcome. We used a TPMT knockdown (kd) model of human Jurkat T-lymphocytes cells to study the effects of treatment with 6-mercaptopurine (6-MP) and 6-thioguanine (6-TG) on proteome and phosphoproteome. We identified thirteen proteins with altered expression and nine proteins with altered phosphorylation signals. Three proteins (THIO, TXD17, and GSTM3) with putative functions in cellular oxidative stress responses were altered by 6-TG treatment and another protein PRDX3 was differentially phosphorylated in TPMT kd cells. Furthermore, reactive oxygen species (ROS) assay results were consistent with a significant induction of oxidative stress by both TPMT knockdown and thiopurine treatments. Immunoblot analyses showed treatment altered expression of key antioxidant enzymes (i.e., SOD2 and catalase) in both wt and kd groups, while SOD1 was downregulated by 6-TG treatment and TPMT knockdown. Collectively, increased oxidative stress might be a mechanism involved in thiopurine induced cytotoxicity and adverse effects (i.e., hepatotoxicity) and an antioxidant cotherapy might help to combat this. Results highlight the significance of oxidative stress in thiopurines' actions and could have important implications for the treatment of IBD patients.
Figures
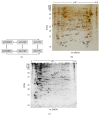
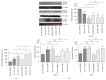
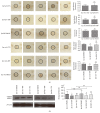
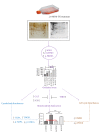
Similar articles
-
Establishment of thiopurine S-methyltransferase gene knockdown in jurkat T-lymphocytes: an in vitro model of TPMT polymorphism.Ther Drug Monit. 2012 Oct;34(5):584-92. doi: 10.1097/FTD.0b013e31826ec4b4. Ther Drug Monit. 2012. PMID: 22972540
-
Differential role of thiopurine methyltransferase in the cytotoxic effects of 6-mercaptopurine and 6-thioguanine on human leukemia cells.Biochem Biophys Res Commun. 2013 Jul 26;437(2):280-6. doi: 10.1016/j.bbrc.2013.06.067. Epub 2013 Jun 27. Biochem Biophys Res Commun. 2013. PMID: 23811272
-
Differing contribution of thiopurine methyltransferase to mercaptopurine versus thioguanine effects in human leukemic cells.Cancer Res. 2001 Aug 1;61(15):5810-6. Cancer Res. 2001. PMID: 11479220
-
Review article: the benefits of pharmacogenetics for improving thiopurine therapy in inflammatory bowel disease.Aliment Pharmacol Ther. 2012 Jan;35(1):15-36. doi: 10.1111/j.1365-2036.2011.04905.x. Epub 2011 Nov 2. Aliment Pharmacol Ther. 2012. PMID: 22050052 Review.
-
Azathioprine and 6-mercaptopurine pharmacogenetics and metabolite monitoring in inflammatory bowel disease.J Gastroenterol Hepatol. 2005 Aug;20(8):1149-57. doi: 10.1111/j.1440-1746.2005.03832.x. J Gastroenterol Hepatol. 2005. PMID: 16048561 Review.
Cited by
-
Photosensitized UVA-Induced Cross-Linking between Human DNA Repair and Replication Proteins and DNA Revealed by Proteomic Analysis.J Proteome Res. 2016 Dec 2;15(12):4612-4623. doi: 10.1021/acs.jproteome.6b00717. Epub 2016 Oct 5. J Proteome Res. 2016. PMID: 27654267 Free PMC article.
-
Hepatic complications induced by immunosuppressants and biologics in inflammatory bowel disease.World J Hepatol. 2017 May 8;9(13):613-626. doi: 10.4254/wjh.v9.i13.613. World J Hepatol. 2017. PMID: 28539989 Free PMC article. Review.
-
Nonenzymatic Serum Antioxidant Capacity in IBD and Its Association with the Severity of Bowel Inflammation and Corticosteroids Treatment.Medicina (Kaunas). 2019 Apr 2;55(4):88. doi: 10.3390/medicina55040088. Medicina (Kaunas). 2019. PMID: 30986917 Free PMC article.
References
-
- Cara C. J., Pena A. S., Sans M., et al. Reviewing the mechanism of action of thiopurine drugs: towards a new paradigm in clinical practice. Medical Science Monitor. 2004;10(11):RA247–RA254. - PubMed
-
- Lee B. W. K., Sun H. G., Zang T., Ju-Kim B., Alfaro J. F., Zhou Z. S. Enzyme-catalyzed transfer of a ketone group from an S-adenosylmethionine analogue: a tool for the functional analysis of methyltransferases. Journal of the American Chemical Society. 2010;132(11):3642–3643. doi: 10.1021/ja908995p. - DOI - PMC - PubMed
MeSH terms
Substances
LinkOut - more resources
Full Text Sources
Other Literature Sources
Research Materials
Miscellaneous