The physiology of protein S-acylation
- PMID: 25834228
- PMCID: PMC4551212
- DOI: 10.1152/physrev.00032.2014
The physiology of protein S-acylation
Abstract
Protein S-acylation, the only fully reversible posttranslational lipid modification of proteins, is emerging as a ubiquitous mechanism to control the properties and function of a diverse array of proteins and consequently physiological processes. S-acylation results from the enzymatic addition of long-chain lipids, most typically palmitate, onto intracellular cysteine residues of soluble and transmembrane proteins via a labile thioester linkage. Addition of lipid results in increases in protein hydrophobicity that can impact on protein structure, assembly, maturation, trafficking, and function. The recent explosion in global S-acylation (palmitoyl) proteomic profiling as a result of improved biochemical tools to assay S-acylation, in conjunction with the recent identification of enzymes that control protein S-acylation and de-acylation, has opened a new vista into the physiological function of S-acylation. This review introduces key features of S-acylation and tools to interrogate this process, and highlights the eclectic array of proteins regulated including membrane receptors, ion channels and transporters, enzymes and kinases, signaling adapters and chaperones, cell adhesion, and structural proteins. We highlight recent findings correlating disruption of S-acylation to pathophysiology and disease and discuss some of the major challenges and opportunities in this rapidly expanding field.
Copyright © 2015 the American Physiological Society.
Figures
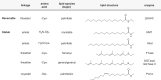
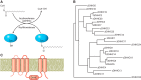
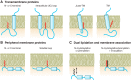
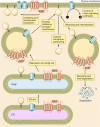
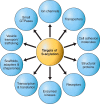
Similar articles
-
Selective Enrichment and Direct Analysis of Protein S-Palmitoylation Sites.J Proteome Res. 2018 May 4;17(5):1907-1922. doi: 10.1021/acs.jproteome.8b00002. Epub 2018 Apr 6. J Proteome Res. 2018. PMID: 29575903 Free PMC article.
-
Chemical Proteomic Analysis of S-Fatty Acylated Proteins and Their Modification Sites.Methods Mol Biol. 2019;2009:45-57. doi: 10.1007/978-1-4939-9532-5_4. Methods Mol Biol. 2019. PMID: 31152394
-
Regulatory effects of post-translational modifications on zDHHC S-acyltransferases.J Biol Chem. 2020 Oct 23;295(43):14640-14652. doi: 10.1074/jbc.REV120.014717. Epub 2020 Aug 17. J Biol Chem. 2020. PMID: 32817054 Free PMC article. Review.
-
Proteome scale characterization of human S-acylated proteins in lipid raft-enriched and non-raft membranes.Mol Cell Proteomics. 2010 Jan;9(1):54-70. doi: 10.1074/mcp.M800448-MCP200. Epub 2009 Oct 2. Mol Cell Proteomics. 2010. PMID: 19801377 Free PMC article.
-
Protein palmitoylation and subcellular trafficking.Biochim Biophys Acta. 2011 Dec;1808(12):2981-94. doi: 10.1016/j.bbamem.2011.07.009. Epub 2011 Jul 23. Biochim Biophys Acta. 2011. PMID: 21819967 Review.
Cited by
-
Regulation of insulin secretion by the post-translational modifications.Front Cell Dev Biol. 2023 Aug 4;11:1217189. doi: 10.3389/fcell.2023.1217189. eCollection 2023. Front Cell Dev Biol. 2023. PMID: 37601108 Free PMC article. Review.
-
The dual roles of RPE65 S-palmitoylation in membrane association and visual cycle function.Sci Rep. 2019 Mar 26;9(1):5218. doi: 10.1038/s41598-019-41501-w. Sci Rep. 2019. PMID: 30914787 Free PMC article.
-
Smad7 palmitoylation by the S-acyltransferase zDHHC17 enhances its inhibitory effect on TGF-β/Smad signaling.J Biol Chem. 2024 Jul;300(7):107462. doi: 10.1016/j.jbc.2024.107462. Epub 2024 Jun 12. J Biol Chem. 2024. PMID: 38876303 Free PMC article.
-
Post-translational palmitoylation controls the voltage gating and lipid raft association of the CALHM1 channel.J Physiol. 2017 Sep 15;595(18):6121-6145. doi: 10.1113/JP274164. Epub 2017 Aug 14. J Physiol. 2017. PMID: 28734079 Free PMC article.
-
Targeting ZDHHC21/FASN axis for the treatment of diffuse large B-cell lymphoma.Leukemia. 2024 Feb;38(2):351-364. doi: 10.1038/s41375-023-02130-5. Epub 2024 Jan 9. Leukemia. 2024. PMID: 38195819 Free PMC article.
References
-
- Adibekian A, Martin BR, Chang JW, Hsu KL, Tsuboi K, Bachovchin DA, Speers AE, Brown SJ, Spicer T, Fernandez-Vega V, Ferguson J, Hodder PS, Rosen H, Cravatt BF. Confirming target engagement for reversible inhibitors in vivo by kinetically tuned activity-based probes. J Am Chem Soc 134: 10345–10348, 2012. - PMC - PubMed
-
- Adlanmerini M, Solinhac R, Abot A, Fabre A, Raymond-Letron I, Guihot AL, Boudou F, Sautier L, Vessières E, Kim SH, Lière P, Fontaine C, Krust A, Chambon P, Katzenellenbogen JA, Gourdy P, Shaul PW, Henrion D, Arnal JF, Lenfant F. Mutation of the palmitoylation site of estrogen receptor α in vivo reveals tissue-specific roles for membrane versus nuclear actions. Proc Natl Acad Sci USA 111: E283–290, 2014. - PMC - PubMed
Publication types
MeSH terms
Substances
Grants and funding
LinkOut - more resources
Full Text Sources
Other Literature Sources
Research Materials