Cargo adaptors: structures illuminate mechanisms regulating vesicle biogenesis
- PMID: 25795254
- PMCID: PMC4475447
- DOI: 10.1016/j.tcb.2015.02.005
Cargo adaptors: structures illuminate mechanisms regulating vesicle biogenesis
Abstract
Cargo adaptors sort transmembrane protein cargos into nascent vesicles by binding directly to their cytosolic domains. Recent studies have revealed previously unappreciated roles for cargo adaptors and regulatory mechanisms governing their function. The adaptor protein (AP)-1 and AP-2 clathrin adaptors switch between open and closed conformations that ensure they function at the right place at the right time. The exomer cargo adaptor has a direct role in remodeling the membrane for vesicle fission. Several different cargo adaptors functioning in distinct trafficking pathways at the Golgi are similarly regulated through bivalent binding to the ADP-ribosylation factor 1 (Arf1) GTPase, potentially enabling regulation by a threshold concentration of Arf1. Taken together, these studies highlight that cargo adaptors do more than just adapt cargos.
Keywords: GTPase; cargo adaptor; membrane trafficking; vesicle.
Copyright © 2015 Elsevier Ltd. All rights reserved.
Figures
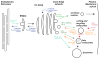
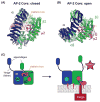
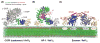
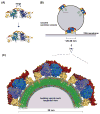
Similar articles
-
Structural basis for membrane binding and remodeling by the exomer secretory vesicle cargo adaptor.Dev Cell. 2014 Sep 8;30(5):610-24. doi: 10.1016/j.devcel.2014.07.014. Dev Cell. 2014. PMID: 25203211 Free PMC article.
-
The exomer cargo adaptor structure reveals a novel GTPase-binding domain.EMBO J. 2012 Nov 5;31(21):4191-203. doi: 10.1038/emboj.2012.268. Epub 2012 Sep 21. EMBO J. 2012. PMID: 23000721 Free PMC article.
-
The clathrin adaptor complexes as a paradigm for membrane-associated allostery.Protein Sci. 2013 May;22(5):517-29. doi: 10.1002/pro.2235. Epub 2013 Mar 18. Protein Sci. 2013. PMID: 23424177 Free PMC article. Review.
-
A role for cargo in Arf-dependent adaptor recruitment.J Biol Chem. 2013 May 24;288(21):14788-804. doi: 10.1074/jbc.M113.453621. Epub 2013 Apr 9. J Biol Chem. 2013. PMID: 23572528 Free PMC article.
-
[GGAs: structure and function of a novel family of clathrin adaptors involved in membrane trafficking between the TGN and endosomes].Seikagaku. 2005 Nov;77(11):1367-81. Seikagaku. 2005. PMID: 16370355 Review. Japanese. No abstract available.
Cited by
-
von Willebrand factor multimerization and the polarity of secretory pathways in endothelial cells.Blood. 2016 Jul 14;128(2):277-85. doi: 10.1182/blood-2015-10-677054. Epub 2016 Apr 22. Blood. 2016. PMID: 27106123 Free PMC article.
-
Plasmodium's fight for survival: escaping elimination while acquiring nutrients.Trends Parasitol. 2022 Jul;38(7):544-557. doi: 10.1016/j.pt.2022.04.004. Epub 2022 May 6. Trends Parasitol. 2022. PMID: 35534377 Free PMC article. Review.
-
Lysosomal Biology and Function: Modern View of Cellular Debris Bin.Cells. 2020 May 4;9(5):1131. doi: 10.3390/cells9051131. Cells. 2020. PMID: 32375321 Free PMC article. Review.
-
Adaptor protein complex-1 (AP-1) is recruited by the HEATR5 protein Laa1 and its co-factor Laa2 in yeast.J Biol Chem. 2019 Jan 25;294(4):1410-1419. doi: 10.1074/jbc.RA118.005253. Epub 2018 Dec 6. J Biol Chem. 2019. PMID: 30523155 Free PMC article.
-
The Arf-GAP Age2 localizes to the late-Golgi via a conserved amphipathic helix.bioRxiv [Preprint]. 2023 Jul 24:2023.07.23.550229. doi: 10.1101/2023.07.23.550229. bioRxiv. 2023. Update in: Mol Biol Cell. 2023 Nov 1;34(12):ar119. doi: 10.1091/mbc.E23-07-0283. PMID: 37546741 Free PMC article. Updated. Preprint.
References
Publication types
MeSH terms
Substances
Grants and funding
LinkOut - more resources
Full Text Sources
Other Literature Sources
Research Materials