RMND5 from Xenopus laevis is an E3 ubiquitin-ligase and functions in early embryonic forebrain development
- PMID: 25793641
- PMCID: PMC4368662
- DOI: 10.1371/journal.pone.0120342
RMND5 from Xenopus laevis is an E3 ubiquitin-ligase and functions in early embryonic forebrain development
Abstract
In Saccharomyces cerevisiae the Gid-complex functions as an ubiquitin-ligase complex that regulates the metabolic switch between glycolysis and gluconeogenesis. In higher organisms six conserved Gid proteins form the CTLH protein-complex with unknown function. Here we show that Rmnd5, the Gid2 orthologue from Xenopus laevis, is an ubiquitin-ligase embedded in a high molecular weight complex. Expression of rmnd5 is strongest in neuronal ectoderm, prospective brain, eyes and ciliated cells of the skin and its suppression results in malformations of the fore- and midbrain. We therefore suggest that Xenopus laevis Rmnd5, as a subunit of the CTLH complex, is a ubiquitin-ligase targeting an unknown factor for polyubiquitination and subsequent proteasomal degradation for proper fore- and midbrain development.
Conflict of interest statement
Figures
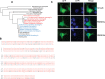
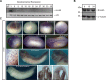
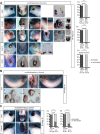
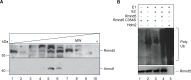
Similar articles
-
Molecular phylogeny of a RING E3 ubiquitin ligase, conserved in eukaryotic cells and dominated by homologous components, the muskelin/RanBPM/CTLH complex.PLoS One. 2013 Oct 15;8(10):e75217. doi: 10.1371/journal.pone.0075217. eCollection 2013. PLoS One. 2013. PMID: 24143168 Free PMC article.
-
Exploring the topology of the Gid complex, the E3 ubiquitin ligase involved in catabolite-induced degradation of gluconeogenic enzymes.J Biol Chem. 2012 Jul 20;287(30):25602-14. doi: 10.1074/jbc.M112.363762. Epub 2012 May 29. J Biol Chem. 2012. PMID: 22645139 Free PMC article.
-
Gid9, a second RING finger protein contributes to the ubiquitin ligase activity of the Gid complex required for catabolite degradation.FEBS Lett. 2011 Dec 15;585(24):3856-61. doi: 10.1016/j.febslet.2011.10.038. Epub 2011 Oct 29. FEBS Lett. 2011. PMID: 22044534
-
Structural and Functional Insights into GID/CTLH E3 Ligase Complexes.Int J Mol Sci. 2022 May 24;23(11):5863. doi: 10.3390/ijms23115863. Int J Mol Sci. 2022. PMID: 35682545 Free PMC article. Review.
-
The Gid-complex: an emerging player in the ubiquitin ligase league.Biol Chem. 2019 Oct 25;400(11):1429-1441. doi: 10.1515/hsz-2019-0139. Biol Chem. 2019. PMID: 30893051 Review.
Cited by
-
WDR26 is a new partner of Axin1 in the canonical Wnt signaling pathway.FEBS Lett. 2016 May;590(9):1291-303. doi: 10.1002/1873-3468.12180. Epub 2016 May 3. FEBS Lett. 2016. PMID: 27098453 Free PMC article.
-
Studies of recombinant TWA1 reveal constitutive dimerization.Biosci Rep. 2017 Jan 13;37(1):BSR20160401. doi: 10.1042/BSR20160401. Print 2017 Feb 28. Biosci Rep. 2017. PMID: 27920276 Free PMC article.
-
miR-590-5p targets RMND5A and promotes migration in pancreatic adenocarcinoma cell lines.Oncol Lett. 2021 Jul;22(1):532. doi: 10.3892/ol.2021.12793. Epub 2021 May 17. Oncol Lett. 2021. PMID: 34079591 Free PMC article.
-
Cryo-EM structures of Gid12-bound GID E3 reveal steric blockade as a mechanism inhibiting substrate ubiquitylation.Nat Commun. 2022 Jun 1;13(1):3041. doi: 10.1038/s41467-022-30803-9. Nat Commun. 2022. PMID: 35650207 Free PMC article.
-
Skraban-Deardorff intellectual disability syndrome-associated mutations in WDR26 impair CTLH E3 complex assembly.FEBS Lett. 2024 May;598(9):978-994. doi: 10.1002/1873-3468.14866. Epub 2024 Apr 4. FEBS Lett. 2024. PMID: 38575527 Free PMC article.
References
-
- Regelmann J, Schule T, Josupeit FS, Horak J, Rose M, Entian KD, et al. Catabolite degradation of fructose-1,6-bisphosphatase in the yeast Saccharomyces cerevisiae: a genome-wide screen identifies eight novel GID genes and indicates the existence of two degradation pathways. Mol Biol Cell. 2003;14(4):1652–63. Epub 2003/04/11. 10.1091/mbc.E02-08-0456 PubMed PMID: . - DOI - PMC - PubMed
-
- Menssen R, Schweiggert J, Schreiner J, Kusevic D, Reuther J, Braun B, et al. Exploring the Topology of the Gid Complex, the E3 Ubiquitin Ligase Involved in Catabolite-induced Degradation of Gluconeogenic Enzymes. J Biol Chem. 2012;287(30):25602–14. Epub 2012/05/31. 10.1074/jbc.M112.363762 PubMed PMID: - DOI - PMC - PubMed
-
- Braun B, Pfirrmann T, Menssen R, Hofmann K, Scheel H, Wolf DH. Gid9, a second RING finger protein contributes to the ubiquitin ligase activity of the Gid complex required for catabolite degradation. FEBS Lett. 2011. Epub 2011/11/03. S0014-5793(11)00789-7 [pii]10.1016/j.febslet.2011.10.038 PubMed PMID: . - DOI - PubMed
-
- Santt O, Pfirrmann T, Braun B, Juretschke J, Kimmig P, Scheel H, et al. The yeast GID complex, a novel ubiquitin ligase (E3) involved in the regulation of carbohydrate metabolism. Mol Biol Cell. 2008;19(8):3323–33. Epub 2008/05/30. E08-03-0328 [pii]10.1091/mbc.E08-03-0328 PubMed PMID: . - DOI - PMC - PubMed
Publication types
MeSH terms
Substances
Grants and funding
LinkOut - more resources
Full Text Sources
Other Literature Sources
Molecular Biology Databases