Precise in-frame integration of exogenous DNA mediated by CRISPR/Cas9 system in zebrafish
- PMID: 25740433
- PMCID: PMC4350073
- DOI: 10.1038/srep08841
Precise in-frame integration of exogenous DNA mediated by CRISPR/Cas9 system in zebrafish
Abstract
The CRISPR/Cas9 system provides a powerful tool for genome editing in various model organisms, including zebrafish. The establishment of targeted gene-disrupted zebrafish (knockouts) is readily achieved by CRISPR/Cas9-mediated genome modification. Recently, exogenous DNA integration into the zebrafish genome via homology-independent DNA repair was reported, but this integration contained various mutations at the junctions of genomic and integrated DNA. Thus, precise genome modification into targeted genomic loci remains to be achieved. Here, we describe efficient, precise CRISPR/Cas9-mediated integration using a donor vector harbouring short homologous sequences (10-40 bp) flanking the genomic target locus. We succeeded in integrating with high efficiency an exogenous mCherry or eGFP gene into targeted genes (tyrosinase and krtt1c19e) in frame. We found the precise in-frame integration of exogenous DNA without backbone vector sequences when Cas9 cleavage sites were introduced at both sides of the left homology arm, the eGFP sequence and the right homology arm. Furthermore, we confirmed that this precise genome modification was heritable. This simple method enables precise targeted gene knock-in in zebrafish.
Figures
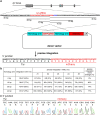
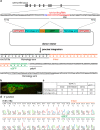
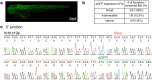
Similar articles
-
CRISPR/Cas9-Mediated Targeted Knockin of Exogenous Reporter Genes in Zebrafish.Methods Mol Biol. 2017;1630:165-173. doi: 10.1007/978-1-4939-7128-2_14. Methods Mol Biol. 2017. PMID: 28643258
-
Site-Specific Integration of Exogenous Genes Using Genome Editing Technologies in Zebrafish.Int J Mol Sci. 2016 May 13;17(5):727. doi: 10.3390/ijms17050727. Int J Mol Sci. 2016. PMID: 27187373 Free PMC article. Review.
-
Practical method for targeted disruption of cilia-related genes by using CRISPR/Cas9-mediated, homology-independent knock-in system.Mol Biol Cell. 2017 Apr 1;28(7):898-906. doi: 10.1091/mbc.E17-01-0051. Epub 2017 Feb 8. Mol Biol Cell. 2017. PMID: 28179459 Free PMC article.
-
Efficient CRISPR/Cas9 genome editing with low off-target effects in zebrafish.Development. 2013 Dec;140(24):4982-7. doi: 10.1242/dev.099085. Epub 2013 Nov 20. Development. 2013. PMID: 24257628
-
Exogenous gene integration mediated by genome editing technologies in zebrafish.Bioengineered. 2017 May 4;8(3):287-295. doi: 10.1080/21655979.2017.1300727. Epub 2017 Mar 8. Bioengineered. 2017. PMID: 28272984 Free PMC article. Review.
Cited by
-
Precise genome editing by homologous recombination.Methods Cell Biol. 2016;135:121-47. doi: 10.1016/bs.mcb.2016.04.008. Epub 2016 May 2. Methods Cell Biol. 2016. PMID: 27443923 Free PMC article.
-
Recent advances in CRISPR-Cas9-based genome insertion technologies.Mol Ther Nucleic Acids. 2024 Feb 5;35(1):102138. doi: 10.1016/j.omtn.2024.102138. eCollection 2024 Mar 12. Mol Ther Nucleic Acids. 2024. PMID: 38379727 Free PMC article. Review.
-
Expanding the CRISPR Toolbox with ErCas12a in Zebrafish and Human Cells.CRISPR J. 2019 Dec;2(6):417-433. doi: 10.1089/crispr.2019.0026. Epub 2019 Nov 19. CRISPR J. 2019. PMID: 31742435 Free PMC article.
-
Speciation and adaptation research meets genome editing.Philos Trans R Soc Lond B Biol Sci. 2022 Jul 18;377(1855):20200516. doi: 10.1098/rstb.2020.0516. Epub 2022 May 30. Philos Trans R Soc Lond B Biol Sci. 2022. PMID: 35634923 Free PMC article. Review.
-
Genetic manipulation of betta fish.Front Genome Ed. 2023 Jul 21;5:1167093. doi: 10.3389/fgeed.2023.1167093. eCollection 2023. Front Genome Ed. 2023. PMID: 37545763 Free PMC article.
References
-
- Bogdanove A. J. & Voytas D. F. TAL effectors: customizable proteins for DNA targeting. Science 333, 1843–1846 (2011). - PubMed
Publication types
MeSH terms
LinkOut - more resources
Full Text Sources
Other Literature Sources
Molecular Biology Databases
Research Materials