Modulation of the chaperone DnaK allosterism by the nucleotide exchange factor GrpE
- PMID: 25739641
- PMCID: PMC4400324
- DOI: 10.1074/jbc.M114.623371
Modulation of the chaperone DnaK allosterism by the nucleotide exchange factor GrpE
Abstract
Hsp70 chaperones comprise two domains, the nucleotide-binding domain (Hsp70NBD), responsible for structural and functional changes in the chaperone, and the substrate-binding domain (Hsp70SBD), involved in substrate interaction. Substrate binding and release in Hsp70 is controlled by the nucleotide state of DnaKNBD, with ATP inducing the open, substrate-receptive DnaKSBD conformation, whereas ADP forces its closure. DnaK cycles between the two conformations through interaction with two cofactors, the Hsp40 co-chaperones (DnaJ in Escherichia coli) induce the ADP state, and the nucleotide exchange factors (GrpE in E. coli) induce the ATP state. X-ray crystallography showed that the GrpE dimer is a nucleotide exchange factor that works by interaction of one of its monomers with DnaKNBD. DnaKSBD location in this complex is debated; there is evidence that it interacts with the GrpE N-terminal disordered region, far from DnaKNBD. Although we confirmed this interaction using biochemical and biophysical techniques, our EM-based three-dimensional reconstruction of the DnaK-GrpE complex located DnaKSBD near DnaKNBD. This apparent discrepancy between the functional and structural results is explained by our finding that the tail region of the GrpE dimer in the DnaK-GrpE complex bends and its tip contacts DnaKSBD, whereas the DnaKNBD-DnaKSBD linker contacts the GrpE helical region. We suggest that these interactions define a more complex role for GrpE in the control of DnaK function.
Keywords: 70-Kilodalton Heat Shock Protein (Hsp70); Chaperone; Chaperone DnaK (DnaK); Electron Microscopy (EM); GrpE; Nucleotide Exchange Factor; Protein Folding.
© 2015 by The American Society for Biochemistry and Molecular Biology, Inc.
Figures
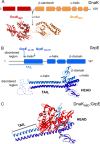
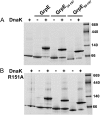
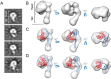
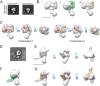
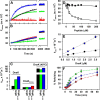
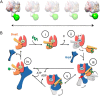
Similar articles
-
New insights into the structure and function of the complex between the Escherichia coli Hsp70, DnaK, and its nucleotide-exchange factor, GrpE.J Biol Chem. 2024 Jan;300(1):105574. doi: 10.1016/j.jbc.2023.105574. Epub 2023 Dec 16. J Biol Chem. 2024. PMID: 38110031 Free PMC article.
-
The heat-sensitive Escherichia coli grpE280 phenotype: impaired interaction of GrpE(G122D) with DnaK.J Mol Biol. 2005 Nov 4;353(4):888-96. doi: 10.1016/j.jmb.2005.08.069. Epub 2005 Sep 20. J Mol Biol. 2005. PMID: 16198374
-
Regulation of ATPase and chaperone cycle of DnaK from Thermus thermophilus by the nucleotide exchange factor GrpE.J Mol Biol. 2001 Feb 2;305(5):1173-83. doi: 10.1006/jmbi.2000.4373. J Mol Biol. 2001. PMID: 11162122
-
GrpE, a nucleotide exchange factor for DnaK.Cell Stress Chaperones. 2003 Fall;8(3):218-24. doi: 10.1379/1466-1268(2003)008<0218:ganeff>2.0.co;2. Cell Stress Chaperones. 2003. PMID: 14984054 Free PMC article. Review.
-
The Escherichia coli chaperones involved in DNA replication.Philos Trans R Soc Lond B Biol Sci. 1993 Mar 29;339(1289):271-7; discussion 277-8. doi: 10.1098/rstb.1993.0025. Philos Trans R Soc Lond B Biol Sci. 1993. PMID: 8098531 Review.
Cited by
-
The nucleotide-bound/substrate-bound conformation of the Mycoplasma genitalium DnaK chaperone.Protein Sci. 2018 May;27(5):1000-1007. doi: 10.1002/pro.3401. Epub 2018 Apr 14. Protein Sci. 2018. PMID: 29520883 Free PMC article.
-
Non-canonical Interactions between Heat Shock Cognate Protein 70 (Hsc70) and Bcl2-associated Anthanogene (BAG) Co-Chaperones Are Important for Client Release.J Biol Chem. 2016 Sep 16;291(38):19848-57. doi: 10.1074/jbc.M116.742502. Epub 2016 Jul 29. J Biol Chem. 2016. PMID: 27474739 Free PMC article.
-
An allosteric inhibitor of bacterial Hsp70 chaperone potentiates antibiotics and mitigates resistance.Cell Chem Biol. 2022 May 19;29(5):854-869.e9. doi: 10.1016/j.chembiol.2021.11.004. Epub 2021 Nov 23. Cell Chem Biol. 2022. PMID: 34818532 Free PMC article.
-
Hsp70 molecular chaperones: multifunctional allosteric holding and unfolding machines.Biochem J. 2019 Jun 14;476(11):1653-1677. doi: 10.1042/BCJ20170380. Biochem J. 2019. PMID: 31201219 Free PMC article. Review.
-
The remarkable multivalency of the Hsp70 chaperones.Cell Stress Chaperones. 2017 Mar;22(2):173-189. doi: 10.1007/s12192-017-0776-y. Epub 2017 Feb 20. Cell Stress Chaperones. 2017. PMID: 28220454 Free PMC article. Review.
References
-
- Hartl F. U., Hayer-Hartl M. (2009) Converging concepts of protein folding in vitro and in vivo. Nat. Struct. Mol. Biol. 16, 574–581 - PubMed
-
- Kim Y. E., Hipp M. S., Bracher A., Hayer-Hartl M., Hartl F. U. (2013) Molecular chaperone functions in protein folding and proteostasis. Annu. Rev. Biochem. 82, 323–355 - PubMed
-
- Young J. C., Barral J. M., Ulrich Hartl F. (2003) More than folding: localized functions of cytosolic chaperones. Trends Biochem. Sci. 28, 541–547 - PubMed
-
- Bukau B., Horwich A. L. (1998) The Hsp70 and Hsp60 chaperone machines. Cell 92, 351–366 - PubMed
Publication types
MeSH terms
Substances
LinkOut - more resources
Full Text Sources
Molecular Biology Databases