A new player at the flagellar motor: FliL controls both motor output and bias
- PMID: 25714720
- PMCID: PMC4358005
- DOI: 10.1128/mBio.02367-14
A new player at the flagellar motor: FliL controls both motor output and bias
Abstract
The bacterial flagellum is driven by a bidirectional rotary motor, which propels bacteria to swim through liquids or swarm over surfaces. While the functions of the major structural and regulatory components of the flagellum are known, the function of the well-conserved FliL protein is not. In Salmonella and Escherichia coli, the absence of FliL leads to a small defect in swimming but complete elimination of swarming. Here, we tracked single motors of these bacteria and found that absence of FliL decreases their speed as well as switching frequency. We demonstrate that FliL interacts strongly with itself, with the MS ring protein FliF, and with the stator proteins MotA and MotB and weakly with the rotor switch protein FliG. These and other experiments show that FliL increases motor output either by recruiting or stabilizing the stators or by increasing their efficiency and contributes additionally to torque generation at higher motor loads. The increased torque enabled by FliL explains why this protein is essential for swarming on an agar surface expected to offer increased resistance to bacterial movement.
Importance: FliL is a well-conserved bacterial flagellar protein whose absence leads to a variety of motility defects, ranging from moderate to complete inhibition of swimming in some bacterial species, inhibition of swarming in others, structural defects that break the flagellar rod during swarming in E. coli and Salmonella, and failure to eject the flagellar filament during the developmental transition of a swimmer to a stalk cell in Caulobacter crescentus. Despite these many phenotypes, a specific function for FliL has remained elusive. Here, we established a central role for FliL at the Salmonella and E. coli motors, where it interacts with both rotor and stator proteins, increases motor output, and contributes to the normal rotational bias of the motor.
Copyright © 2015 Partridge et al.
Figures
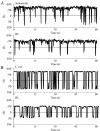
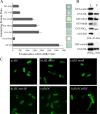
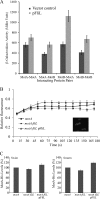
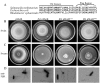
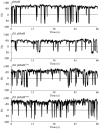
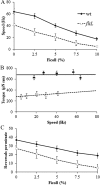
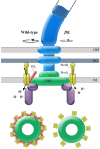
Similar articles
-
FliL is essential for swarming: motor rotation in absence of FliL fractures the flagellar rod in swarmer cells of Salmonella enterica.Mol Microbiol. 2008 Apr;68(2):328-41. doi: 10.1111/j.1365-2958.2008.06170.x. Epub 2008 Feb 19. Mol Microbiol. 2008. PMID: 18284590
-
Flagellar motor remodeling during swarming requires FliL.Mol Microbiol. 2023 Nov;120(5):670-683. doi: 10.1111/mmi.15148. Epub 2023 Sep 7. Mol Microbiol. 2023. PMID: 37675594 Free PMC article.
-
Structure of Vibrio FliL, a New Stomatin-like Protein That Assists the Bacterial Flagellar Motor Function.mBio. 2019 Mar 19;10(2):e00292-19. doi: 10.1128/mBio.00292-19. mBio. 2019. PMID: 30890608 Free PMC article.
-
Flagellar protein FliL: A many-splendored thing.Mol Microbiol. 2024 Oct;122(4):447-454. doi: 10.1111/mmi.15301. Epub 2024 Aug 3. Mol Microbiol. 2024. PMID: 39096095 Review.
-
Structure and function of the bi-directional bacterial flagellar motor.Biomolecules. 2014 Feb 18;4(1):217-34. doi: 10.3390/biom4010217. Biomolecules. 2014. PMID: 24970213 Free PMC article. Review.
Cited by
-
Characterization of FliL Proteins in Bradyrhizobium diazoefficiens: Lateral FliL Supports Swimming Motility, and Subpolar FliL Modulates the Lateral Flagellar System.J Bacteriol. 2020 Feb 11;202(5):e00708-19. doi: 10.1128/JB.00708-19. Print 2020 Feb 11. J Bacteriol. 2020. PMID: 31843800 Free PMC article.
-
FliL Differentially Interacts with Two Stator Systems To Regulate Flagellar Motor Output in Pseudomonas aeruginosa.Appl Environ Microbiol. 2022 Nov 22;88(22):e0153922. doi: 10.1128/aem.01539-22. Epub 2022 Oct 26. Appl Environ Microbiol. 2022. PMID: 36286538 Free PMC article.
-
FliL ring enhances the function of periplasmic flagella.Proc Natl Acad Sci U S A. 2022 Mar 15;119(11):e2117245119. doi: 10.1073/pnas.2117245119. Epub 2022 Mar 7. Proc Natl Acad Sci U S A. 2022. PMID: 35254893 Free PMC article.
-
Flagellar motor remodeling during swarming requires FliL.bioRxiv [Preprint]. 2023 Jul 14:2023.07.14.549092. doi: 10.1101/2023.07.14.549092. bioRxiv. 2023. Update in: Mol Microbiol. 2023 Nov;120(5):670-683. doi: 10.1111/mmi.15148 PMID: 37503052 Free PMC article. Updated. Preprint.
-
Flagellar Perturbations Activate Adhesion through Two Distinct Pathways in Caulobacter crescentus.mBio. 2021 Feb 9;12(1):e03266-20. doi: 10.1128/mBio.03266-20. mBio. 2021. PMID: 33563824 Free PMC article.
References
Publication types
MeSH terms
Substances
Grants and funding
LinkOut - more resources
Full Text Sources
Molecular Biology Databases