Oxidized glutathione (GSSG) inhibits epithelial sodium channel activity in primary alveolar epithelial cells
- PMID: 25713321
- PMCID: PMC4888545
- DOI: 10.1152/ajplung.00213.2014
Oxidized glutathione (GSSG) inhibits epithelial sodium channel activity in primary alveolar epithelial cells
Abstract
Amiloride-sensitive epithelial Na(+) channels (ENaC) regulate fluid balance in the alveoli and are regulated by oxidative stress. Since glutathione (GSH) is the predominant antioxidant in the lungs, we proposed that changes in glutathione redox potential (Eh) would alter cell signaling and have an effect on ENaC open probability (Po). In the present study, we used single channel patch-clamp recordings to examine the effect of oxidative stress, via direct application of glutathione disulfide (GSSG), on ENaC activity. We found a linear decrease in ENaC activity as the GSH/GSSG Eh became less negative (n = 21; P < 0.05). Treatment of 400 μM GSSG to the cell bath significantly decreased ENaC Po from 0.39 ± 0.06 to 0.13 ± 0.05 (n = 8; P < 0.05). Likewise, back-filling recording electrodes with 400 μM GSSG reduced ENaC Po from 0.32 ± 0.08 to 0.17 ± 0.05 (n = 10; P < 0.05), thus implicating GSSG as an important regulatory factor. Biochemical assays indicated that oxidizing potentials promote S-glutathionylation of ENaC and irreversible oxidation of cysteine residues with N-ethylmaleimide blocked the effects of GSSG on ENaC Po. Additionally, real-time imaging studies showed that GSSG impairs alveolar fluid clearance in vivo as opposed to GSH, which did not impair clearance. Taken together, these data show that glutathione Eh is an important determinant of alveolar fluid clearance in vivo.
Keywords: Cys thiol; alveolar flooding; oxidative stress; redox potential.
Copyright © 2015 the American Physiological Society.
Figures
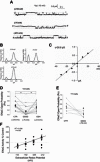
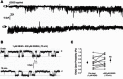
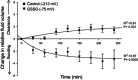
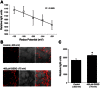
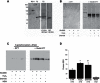
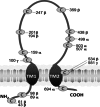
Similar articles
-
Enhanced epithelial sodium channel activity in neonatal Scnn1b mouse lung attenuates high oxygen-induced lung injury.Am J Physiol Lung Cell Mol Physiol. 2021 Jul 1;321(1):L29-L41. doi: 10.1152/ajplung.00538.2020. Epub 2021 May 5. Am J Physiol Lung Cell Mol Physiol. 2021. PMID: 33949206 Free PMC article.
-
Hypoxia-induced inhibition of epithelial Na(+) channels in the lung. Role of Nedd4-2 and the ubiquitin-proteasome pathway.Am J Respir Cell Mol Biol. 2014 Mar;50(3):526-37. doi: 10.1165/rcmb.2012-0518OC. Am J Respir Cell Mol Biol. 2014. PMID: 24093724
-
Alveolar nonselective channels are ASIC1a/α-ENaC channels and contribute to AFC.Am J Physiol Lung Cell Mol Physiol. 2017 Jun 1;312(6):L797-L811. doi: 10.1152/ajplung.00379.2016. Epub 2017 Mar 10. Am J Physiol Lung Cell Mol Physiol. 2017. PMID: 28283476 Free PMC article.
-
Importance of ENaC-mediated sodium transport in alveolar fluid clearance using genetically-engineered mice.Cell Physiol Biochem. 2010;25(1):63-70. doi: 10.1159/000272051. Epub 2009 Dec 22. Cell Physiol Biochem. 2010. PMID: 20054145 Review.
-
Regulation of Na+ channels in lung alveolar type II epithelial cells.Proc Am Thorac Soc. 2004;1(1):10-6. doi: 10.1513/pats.2306008. Proc Am Thorac Soc. 2004. PMID: 16113405 Review.
Cited by
-
RAGE-induced changes in the proteome of alveolar epithelial cells.J Proteomics. 2018 Apr 15;177:11-20. doi: 10.1016/j.jprot.2018.02.010. Epub 2018 Feb 12. J Proteomics. 2018. PMID: 29448054 Free PMC article.
-
Influenza virus infection alters ion channel function of airway and alveolar cells: mechanisms and physiological sequelae.Am J Physiol Lung Cell Mol Physiol. 2017 Nov 1;313(5):L845-L858. doi: 10.1152/ajplung.00244.2017. Epub 2017 Aug 3. Am J Physiol Lung Cell Mol Physiol. 2017. PMID: 28775098 Free PMC article. Review.
-
Glutathione: A Key Regulator of Extracellular Matrix and Cell Death in Intervertebral Disc Degeneration.Mediators Inflamm. 2024 Oct 1;2024:4482642. doi: 10.1155/2024/4482642. eCollection 2024. Mediators Inflamm. 2024. PMID: 39403548 Free PMC article. Review.
-
Oxidative stress, autophagy and airway ion transport.Am J Physiol Cell Physiol. 2019 Jan 1;316(1):C16-C32. doi: 10.1152/ajpcell.00341.2018. Epub 2018 Oct 10. Am J Physiol Cell Physiol. 2019. PMID: 30303690 Free PMC article. Review.
-
Cytokine-Ion Channel Interactions in Pulmonary Inflammation.Front Immunol. 2018 Jan 4;8:1644. doi: 10.3389/fimmu.2017.01644. eCollection 2017. Front Immunol. 2018. PMID: 29354115 Free PMC article. Review.
References
-
- Asayama K, Yokota S, Dobashi K, Kawada Y, Nakane T, Kawaoi A, Nakazawa S. Immunolocalization of cellular glutathione peroxidase in adult rat lungs and quantitative analysis after postembedding immunogold labeling. Histochem Cell Biol 105: 383–389, 1996. - PubMed
-
- Ashfaq S, Abramson JL, Jones DP, Rhodes SD, Weintraub WS, Hooper WC, Vaccarino V, Harrison DG, Quyyumi AA. The relationship between plasma levels of oxidized and reduced thiols and early atherosclerosis in healthy adults. J Am Coll Cardiol 47: 1005–1011, 2006. - PubMed
-
- Bao HF, Zhang ZR, Liang YY, Ma JJ, Eaton DC, Ma HP. Ceramide mediates inhibition of the renal epithelial sodium channel by tumor necrosis factor-alpha through protein kinase C. Am J Physiol Renal Physiol 293: F1178–F1186, 2007. - PubMed
-
- Beigi F, Gonzalez DR, Minhas KM, Sun QA, Foster MW, Khan SA, Treuer AV, Dulce RA, Harrison RW, Saraiva RM, Premer C, Schulman IH, Stamler JS, Hare JM. Dynamic denitrosylation via S-nitrosoglutathione reductase regulates cardiovascular function. Proc Natl Acad Sci USA 109: 4314–4319, 2012. - PMC - PubMed
MeSH terms
Substances
Grants and funding
LinkOut - more resources
Full Text Sources
Other Literature Sources