Alu RNA regulates the cellular pool of active ribosomes by targeted delivery of SRP9/14 to 40S subunits
- PMID: 25697503
- PMCID: PMC4357698
- DOI: 10.1093/nar/gkv048
Alu RNA regulates the cellular pool of active ribosomes by targeted delivery of SRP9/14 to 40S subunits
Abstract
The human genome contains about 1.5 million Alu elements, which are transcribed into Alu RNAs by RNA polymerase III. Their expression is upregulated following stress and viral infection, and they associate with the SRP9/14 protein dimer in the cytoplasm forming Alu RNPs. Using cell-free translation, we have previously shown that Alu RNPs inhibit polysome formation. Here, we describe the mechanism of Alu RNP-mediated inhibition of translation initiation and demonstrate its effect on translation of cellular and viral RNAs. Both cap-dependent and IRES-mediated initiation is inhibited. Inhibition involves direct binding of SRP9/14 to 40S ribosomal subunits and requires Alu RNA as an assembly factor but its continuous association with 40S subunits is not required for inhibition. Binding of SRP9/14 to 40S prevents 48S complex formation by interfering with the recruitment of mRNA to 40S subunits. In cells, overexpression of Alu RNA decreases translation of reporter mRNAs and this effect is alleviated with a mutation that reduces its affinity for SRP9/14. Alu RNPs also inhibit the translation of cellular mRNAs resuming translation after stress and of viral mRNAs suggesting a role of Alu RNPs in adapting the translational output in response to stress and viral infection.
© The Author(s) 2015. Published by Oxford University Press on behalf of Nucleic Acids Research.
Figures
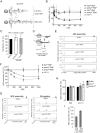
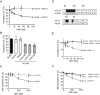
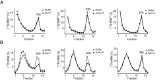
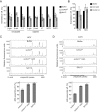
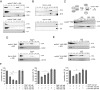
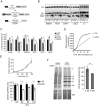
Similar articles
-
Direct binding of the Alu binding protein dimer SRP9/14 to 40S ribosomal subunits promotes stress granule formation and is regulated by Alu RNA.Nucleic Acids Res. 2014;42(17):11203-17. doi: 10.1093/nar/gku822. Epub 2014 Sep 8. Nucleic Acids Res. 2014. PMID: 25200073 Free PMC article.
-
Alu RNP and Alu RNA regulate translation initiation in vitro.Nucleic Acids Res. 2006 May 8;34(8):2374-85. doi: 10.1093/nar/gkl246. Print 2006. Nucleic Acids Res. 2006. PMID: 16682445 Free PMC article.
-
The SRP9/14 subunit of the human signal recognition particle binds to a variety of Alu-like RNAs and with higher affinity than its mouse homolog.Nucleic Acids Res. 1997 Jan 15;25(2):318-26. doi: 10.1093/nar/25.2.318. Nucleic Acids Res. 1997. PMID: 9016560 Free PMC article.
-
Targeting internal ribosome entry site (IRES)-mediated translation to block hepatitis C and other RNA viruses.FEMS Microbiol Lett. 2004 May 15;234(2):189-99. doi: 10.1016/j.femsle.2004.03.045. FEMS Microbiol Lett. 2004. PMID: 15135522 Review.
-
Translation initiation by factor-independent binding of eukaryotic ribosomes to internal ribosomal entry sites.C R Biol. 2005 Jul;328(7):589-605. doi: 10.1016/j.crvi.2005.02.004. C R Biol. 2005. PMID: 15992743 Review.
Cited by
-
ALU non-B-DNA conformations, flipons, binary codes and evolution.R Soc Open Sci. 2020 Jun 3;7(6):200222. doi: 10.1098/rsos.200222. eCollection 2020 Jun. R Soc Open Sci. 2020. PMID: 32742689 Free PMC article. Review.
-
Genetic and clinical predictors of CD4 lymphocyte recovery during suppressive antiretroviral therapy: Whole exome sequencing and antiretroviral therapy response phenotypes.PLoS One. 2019 Aug 15;14(8):e0219201. doi: 10.1371/journal.pone.0219201. eCollection 2019. PLoS One. 2019. PMID: 31415590 Free PMC article.
-
The Pol III transcriptome: Basic features, recurrent patterns, and emerging roles in cancer.Wiley Interdiscip Rev RNA. 2023 Sep-Oct;14(5):e1782. doi: 10.1002/wrna.1782. Epub 2023 Feb 8. Wiley Interdiscip Rev RNA. 2023. PMID: 36754845 Free PMC article. Review.
-
The L1-dependant and Pol III transcribed Alu retrotransposon, from its discovery to innate immunity.Mol Biol Rep. 2021 Mar;48(3):2775-2789. doi: 10.1007/s11033-021-06258-4. Epub 2021 Mar 16. Mol Biol Rep. 2021. PMID: 33725281 Free PMC article. Review.
-
Human rDNA and Cancer.Cells. 2021 Dec 8;10(12):3452. doi: 10.3390/cells10123452. Cells. 2021. PMID: 34943960 Free PMC article. Review.
References
-
- Aitken C.E., Lorsch J.R. A mechanistic overview of translation initiation in eukaryotes. Nat. Struct. Mol. Biol. 2012;19:568–576. - PubMed
Publication types
MeSH terms
Substances
LinkOut - more resources
Full Text Sources
Other Literature Sources
Molecular Biology Databases
Research Materials
Miscellaneous