Rice stripe tenuivirus nonstructural protein 3 hijacks the 26S proteasome of the small brown planthopper via direct interaction with regulatory particle non-ATPase subunit 3
- PMID: 25653432
- PMCID: PMC4442352
- DOI: 10.1128/JVI.03055-14
Rice stripe tenuivirus nonstructural protein 3 hijacks the 26S proteasome of the small brown planthopper via direct interaction with regulatory particle non-ATPase subunit 3
Abstract
The ubiquitin/26S proteasome system plays a vital role in regulating host defenses against pathogens. Previous studies have highlighted different roles for the ubiquitin/26S proteasome in defense during virus infection in both mammals and plants, but their role in the vectors that transmit those viruses is still unclear. In this study, we determined that the 26S proteasome is present in the small brown planthopper (SBPH) (Laodelphax striatellus) and has components similar to those in plants and mammals. There was an increase in the accumulation of Rice stripe virus (RSV) in the transmitting vector SBPH after disrupting the 26S proteasome, indicating that the SBPH 26S proteasome plays a role in defense against RSV infection by regulating RSV accumulation. Yeast two-hybrid analysis determined that a subunit of the 26S proteasome, named RPN3, could interact with RSV NS3. Transient overexpression of RPN3 had no effect on the RNA silencing suppressor activity of RSV NS3. However, NS3 could inhibit the ability of SBPH rpn3 to complement an rpn3 mutation in yeast. Our findings also indicate that the direct interaction between RPN3 and NS3 was responsible for inhibiting the complementation ability of RPN3. In vivo, we found an accumulation of ubiquitinated protein in SBPH tissues where the RSV titer was high, and silencing of rpn3 resulted in malfunction of the SBPH proteasome-mediated proteolysis. Consequently, viruliferous SBPH in which RPN3 was repressed transmitted the virus more effectively as a result of higher accumulation of RSV. Our results suggest that the RSV NS3 protein is able to hijack the 26S proteasome in SBPH via a direct interaction with the RPN3 subunit to attenuate the host defense response.
Importance: We show, for the first time, that the 26S proteasome components are present in the small brown planthopper and play a role in defense against its vectored plant virus (RSV). In turn, RSV encodes a protein that subverts the SBPH 26S proteasome via direct interaction with the 26S proteasome subunit RPN3. Our results imply that the molecular arms race observed in plant hosts can be extended to the insect vector that transmits those viruses.
Copyright © 2015, American Society for Microbiology. All Rights Reserved.
Figures
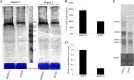
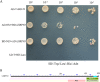
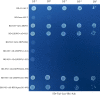
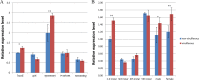
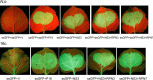
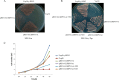
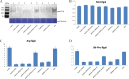
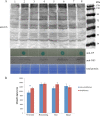
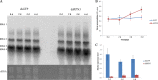
Similar articles
-
An insect cell line derived from the small brown planthopper supports replication of rice stripe virus, a tenuivirus.J Gen Virol. 2013 Jun;94(Pt 6):1421-1425. doi: 10.1099/vir.0.050104-0. Epub 2013 Mar 6. J Gen Virol. 2013. PMID: 23468422
-
Silencing suppressors of rice black-streaked dwarf virus and rice stripe virus hijack the 26S proteasome of Laodelphax striatellus to facilitate virus accumulation and transmission.Pest Manag Sci. 2022 Jul;78(7):2940-2951. doi: 10.1002/ps.6918. Epub 2022 May 12. Pest Manag Sci. 2022. PMID: 35439336
-
Ribosomal protein L18 is an essential factor that promote rice stripe virus accumulation in small brown planthopper.Virus Res. 2018 Mar 2;247:15-20. doi: 10.1016/j.virusres.2018.01.011. Epub 2018 Jan 31. Virus Res. 2018. PMID: 29374519
-
The small brown planthopper (Laodelphax striatellus) as a vector of the rice stripe virus.Arch Insect Biochem Physiol. 2023 Feb;112(2):e21992. doi: 10.1002/arch.21992. Epub 2022 Dec 27. Arch Insect Biochem Physiol. 2023. PMID: 36575628 Review.
-
Plant Virus Infection and the Ubiquitin Proteasome Machinery: Arms Race along the Endoplasmic Reticulum.Viruses. 2016 Nov 19;8(11):314. doi: 10.3390/v8110314. Viruses. 2016. PMID: 27869775 Free PMC article. Review.
Cited by
-
Applications of Proteomic Tools to Study Insect Vector-Plant Virus Interactions.Life (Basel). 2020 Aug 7;10(8):143. doi: 10.3390/life10080143. Life (Basel). 2020. PMID: 32784674 Free PMC article. Review.
-
Rice stripe virus nonstructural protein 3 suppresses plant defence responses mediated by the MEL-SHMT1 module.Mol Plant Pathol. 2023 Nov;24(11):1359-1369. doi: 10.1111/mpp.13373. Epub 2023 Jul 5. Mol Plant Pathol. 2023. PMID: 37404045 Free PMC article.
-
The Bunyavirales: The Plant-Infecting Counterparts.Viruses. 2021 May 6;13(5):842. doi: 10.3390/v13050842. Viruses. 2021. PMID: 34066457 Free PMC article. Review.
-
The c-Jun N-terminal kinase pathway of a vector insect is activated by virus capsid protein and promotes viral replication.Elife. 2017 Jul 18;6:e26591. doi: 10.7554/eLife.26591. Elife. 2017. PMID: 28716183 Free PMC article.
-
A class of independently evolved transcriptional repressors in plant RNA viruses facilitates viral infection and vector feeding.Proc Natl Acad Sci U S A. 2021 Mar 16;118(11):e2016673118. doi: 10.1073/pnas.2016673118. Proc Natl Acad Sci U S A. 2021. PMID: 33836579 Free PMC article.
References
-
- Park HM, Choi MS, Kwak DY, Lee BC, Lee J H, Kim MK, Kim YG, Shin DB, Park SK, Kim YH. 2012. Suppression of NS3 and MP is important for the stable inheritance of RNAi-mediated Rice stripe virus (RSV) resistance obtained by targeting the fully complementary RSV-CP gene. Mol Cells 33:43–51. doi:10.1007/s10059-012-2185-5. - DOI - PMC - PubMed
-
- Kisimoto R. 1965. On the transovarial passage of the Rice stripe virus through the small brown planthopper, Laodelphax striatelllus Fallen, p 73–90. In Conference on relationships between arthropods and plantpathogenic viruses, Tokyo, Japan.
Publication types
MeSH terms
Substances
LinkOut - more resources
Full Text Sources