Myocardin regulates vascular smooth muscle cell inflammatory activation and disease
- PMID: 25614278
- PMCID: PMC4390125
- DOI: 10.1161/ATVBAHA.114.305218
Myocardin regulates vascular smooth muscle cell inflammatory activation and disease
Abstract
Objective: Atherosclerosis, the cause of 50% of deaths in westernized societies, is widely regarded as a chronic vascular inflammatory disease. Vascular smooth muscle cell (VSMC) inflammatory activation in response to local proinflammatory stimuli contributes to disease progression and is a pervasive feature in developing atherosclerotic plaques. Therefore, it is of considerable therapeutic importance to identify mechanisms that regulate the VSMC inflammatory response.
Approach and results: We report that myocardin, a powerful myogenic transcriptional coactivator, negatively regulates VSMC inflammatory activation and vascular disease. Myocardin levels are reduced during atherosclerosis, in association with phenotypic switching of smooth muscle cells. Myocardin deficiency accelerates atherogenesis in hypercholesterolemic apolipoprotein E(-/-) mice. Conversely, increased myocardin expression potently abrogates the induction of an array of inflammatory cytokines, chemokines, and adhesion molecules in VSMCs. Expression of myocardin in VSMCs reduces lipid uptake, macrophage interaction, chemotaxis, and macrophage-endothelial tethering in vitro, and attenuates monocyte accumulation within developing lesions in vivo. These results demonstrate that endogenous levels of myocardin are a critical regulator of vessel inflammation.
Conclusions: We propose myocardin as a guardian of the contractile, noninflammatory VSMC phenotype, with loss of myocardin representing a critical permissive step in the process of phenotypic transition and inflammatory activation, at the onset of vascular disease.
Keywords: atherosclerosis; inflammation; myocardin; smooth muscle.
© 2015 American Heart Association, Inc.
Figures
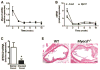
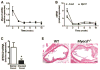
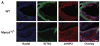
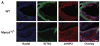
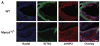
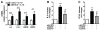
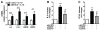
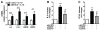
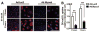
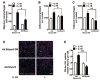
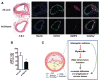
Similar articles
-
Myocardin regulates vascular response to injury through miR-24/-29a and platelet-derived growth factor receptor-β.Arterioscler Thromb Vasc Biol. 2013 Oct;33(10):2355-65. doi: 10.1161/ATVBAHA.112.301000. Epub 2013 Jul 3. Arterioscler Thromb Vasc Biol. 2013. PMID: 23825366
-
c-Myb Regulates Proliferation and Differentiation of Adventitial Sca1+ Vascular Smooth Muscle Cell Progenitors by Transactivation of Myocardin.Arterioscler Thromb Vasc Biol. 2016 Jul;36(7):1367-76. doi: 10.1161/ATVBAHA.115.307116. Epub 2016 May 12. Arterioscler Thromb Vasc Biol. 2016. PMID: 27174098
-
Cholesterol loading reprograms the microRNA-143/145-myocardin axis to convert aortic smooth muscle cells to a dysfunctional macrophage-like phenotype.Arterioscler Thromb Vasc Biol. 2015 Mar;35(3):535-46. doi: 10.1161/ATVBAHA.114.304029. Epub 2015 Jan 8. Arterioscler Thromb Vasc Biol. 2015. PMID: 25573853 Free PMC article.
-
Myocardin: A novel player in atherosclerosis.Atherosclerosis. 2017 Feb;257:266-278. doi: 10.1016/j.atherosclerosis.2016.12.002. Epub 2016 Dec 1. Atherosclerosis. 2017. PMID: 28012646 Review.
-
Emerging Roles of Tumor Necrosis Factor-Stimulated Gene-6 in the Pathophysiology and Treatment of Atherosclerosis.Int J Mol Sci. 2018 Feb 5;19(2):465. doi: 10.3390/ijms19020465. Int J Mol Sci. 2018. PMID: 29401724 Free PMC article. Review.
Cited by
-
The short and long of noncoding sequences in the control of vascular cell phenotypes.Cell Mol Life Sci. 2015 Sep;72(18):3457-88. doi: 10.1007/s00018-015-1936-9. Epub 2015 May 29. Cell Mol Life Sci. 2015. PMID: 26022065 Free PMC article. Review.
-
Targeting non-coding RNAs in unstable atherosclerotic plaques: Mechanism, regulation, possibilities, and limitations.Int J Biol Sci. 2021 Aug 3;17(13):3413-3427. doi: 10.7150/ijbs.62506. eCollection 2021. Int J Biol Sci. 2021. PMID: 34512156 Free PMC article. Review.
-
Triglyceride-rich lipoproteins and cardiovascular diseases.Front Endocrinol (Lausanne). 2024 May 31;15:1409653. doi: 10.3389/fendo.2024.1409653. eCollection 2024. Front Endocrinol (Lausanne). 2024. PMID: 38883601 Free PMC article. Review.
-
Differentiated Smooth Muscle Cells Generate a Subpopulation of Resident Vascular Progenitor Cells in the Adventitia Regulated by Klf4.Circ Res. 2017 Jan 20;120(2):296-311. doi: 10.1161/CIRCRESAHA.116.309322. Epub 2016 Nov 9. Circ Res. 2017. PMID: 27834190 Free PMC article.
-
Selective expression of TSPAN2 in vascular smooth muscle is independently regulated by TGF-β1/SMAD and myocardin/serum response factor.FASEB J. 2017 Jun;31(6):2576-2591. doi: 10.1096/fj.201601021R. Epub 2017 Mar 3. FASEB J. 2017. PMID: 28258189 Free PMC article.
References
-
- Dahlöf B. Cardiovascular disease risk factors: epidemiology and risk assessment. Am J Cardiol. 2010;105:3A–9A. - PubMed
-
- Celermajer DS, Chow CK, Marijon E, Anstey NM, Woo KS. Cardiovascular disease in the developing world: prevalences, patterns, and the potential of early disease detection. J Am Coll Cardiol. 2012;60:1207–1216. - PubMed
-
- Owens GK, Kumar MS, Wamhoff BR. Molecular regulation of vascular smooth muscle cell differentiation in development and disease. Physiol Rev. 2004;84:767–801. - PubMed
Publication types
MeSH terms
Substances
Grants and funding
- R01 HL117907/HL/NHLBI NIH HHS/United States
- FS/13/29/30024/BHF_/British Heart Foundation/United Kingdom
- PG/10/007/28184/BHF_/British Heart Foundation/United Kingdom
- RG/13/14/30314/BHF_/British Heart Foundation/United Kingdom
- 086799/Z/08/Z/WT_/Wellcome Trust/United Kingdom
- R01 HL132574/HL/NHLBI NIH HHS/United States
- RG/13/4/30107/BHF_/British Heart Foundation/United Kingdom
- Wellcome Trust/United Kingdom
- G1000847/MRC_/Medical Research Council/United Kingdom
- RG/08/009/25841/BHF_/British Heart Foundation/United Kingdom
- HL-117907/HL/NHLBI NIH HHS/United States
- G0800784/MRC_/Medical Research Council/United Kingdom
LinkOut - more resources
Full Text Sources
Medical
Molecular Biology Databases