DNA/RNA Detection Using DNA-Templated Few-Atom Silver Nanoclusters
- PMID: 25586126
- PMCID: PMC4263537
- DOI: 10.3390/bios3020185
DNA/RNA Detection Using DNA-Templated Few-Atom Silver Nanoclusters
Abstract
DNA-templated few-atom silver nanoclusters (DNA/Ag NCs) are a new class of organic/inorganic composite nanomaterials whose fluorescence emission can be tuned throughout the visible and near-IR range by simply programming the template sequences. Compared to organic dyes, DNA/Ag NCs can be brighter and more photostable. Compared to quantum dots, DNA/Ag NCs are smaller, less prone to blinking on long timescales, and do not have a toxic core. The preparation of DNA/Ag NCs is simple and there is no need to remove excess precursors as these precursors are non-fluorescent. Our recent discovery of the fluorogenic and color switching properties of DNA/Ag NCs have led to the invention of new molecular probes, termed NanoCluster Beacons (NCBs), for DNA detection, with the capability to differentiate single-nucleotide polymorphisms by emission colors. NCBs are inexpensive, easy to prepare, and compatible with commercial DNA synthesizers. Many other groups have also explored and taken advantage of the environment sensitivities of DNA/Ag NCs in creating new tools for DNA/RNA detection and single-nucleotide polymorphism identification. In this review, we summarize the recent trends in the use of DNA/Ag NCs for developing DNA/RNA sensors.
Figures
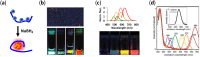
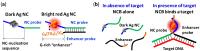
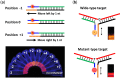
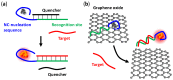
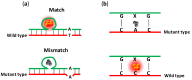
Similar articles
-
DNA Templated Metal Nanoclusters: From Emergent Properties to Unique Applications.Acc Chem Res. 2018 Nov 20;51(11):2756-2763. doi: 10.1021/acs.accounts.8b00366. Epub 2018 Oct 19. Acc Chem Res. 2018. PMID: 30339358
-
A complementary palette of NanoCluster Beacons.ACS Nano. 2014 Oct 28;8(10):10150-60. doi: 10.1021/nn505338e. Epub 2014 Oct 17. ACS Nano. 2014. PMID: 25299363 Free PMC article.
-
A DNA--silver nanocluster probe that fluoresces upon hybridization.Nano Lett. 2010 Aug 11;10(8):3106-10. doi: 10.1021/nl101773c. Nano Lett. 2010. PMID: 20698624
-
Fluorescent silver nanoclusters as DNA probes.Nanoscale. 2013 Sep 21;5(18):8443-61. doi: 10.1039/c3nr01601c. Nanoscale. 2013. PMID: 23828021 Review.
-
Anion-templated silver nanoclusters: precise synthesis and geometric structure.Sci Technol Adv Mater. 2023 May 25;24(1):2203832. doi: 10.1080/14686996.2023.2203832. eCollection 2023. Sci Technol Adv Mater. 2023. PMID: 37251258 Free PMC article. Review.
Cited by
-
Micro and nanotechnologies enhanced biomolecular sensing.Biosensors (Basel). 2013 Jul 5;3(3):283-5. doi: 10.3390/bios3030283. Biosensors (Basel). 2013. PMID: 25586258 Free PMC article.
-
DNA as UV light-harvesting antenna.Nucleic Acids Res. 2018 Apr 20;46(7):3543-3551. doi: 10.1093/nar/gkx1185. Nucleic Acids Res. 2018. PMID: 29186575 Free PMC article.
-
Split Spinach Aptamer for Highly Selective Recognition of DNA and RNA at Ambient Temperatures.Chembiochem. 2016 Sep 2;17(17):1589-92. doi: 10.1002/cbic.201600323. Epub 2016 Jul 15. Chembiochem. 2016. PMID: 27305425 Free PMC article.
-
Detection of p53 Gene Mutation (Single-Base Mismatch) Using a Fluorescent Silver Nanoclusters.J Fluoresc. 2017 Jul;27(4):1443-1448. doi: 10.1007/s10895-017-2083-5. Epub 2017 Apr 13. J Fluoresc. 2017. PMID: 28405933
-
Near-infrared silver cluster optically signaling oligonucleotide hybridization and assembling two DNA hosts.Anal Chem. 2014 Sep 16;86(18):9220-8. doi: 10.1021/ac502192w. Epub 2014 Sep 4. Anal Chem. 2014. PMID: 25157472 Free PMC article.
References
-
- Sozzi G., Conte D., Mariani L., Vullo S.L., Roz L., Lombardo C., Pierotti M.A., Tavecchio L. Analysis of circulating tumor DNA in plasma at diagnosis and during follow-up of lung cancer patients. Canc. Res. 2001;61:4675–4678. - PubMed
Publication types
LinkOut - more resources
Full Text Sources
Other Literature Sources