Dynamics of miRNA driven feed-forward loop depends upon miRNA action mechanisms
- PMID: 25563303
- PMCID: PMC4303955
- DOI: 10.1186/1471-2164-15-S12-S9
Dynamics of miRNA driven feed-forward loop depends upon miRNA action mechanisms
Abstract
Background: We perform the theoretical analysis of a gene network sub-system, composed of a feed-forward loop, in which the upstream transcription factor regulates the target gene via two parallel pathways: directly, and via interaction with miRNA.
Results: As the molecular mechanisms of miRNA action are not clear so far, we elaborate three mathematical models, in which miRNA either represses translation of its target or promotes target mRNA degradation, or is not re-used, but degrades along with target mRNA. We examine the feed-forward loop dynamics quantitatively at the whole time interval of cell cycle. We rigorously proof the uniqueness of solutions to the models and obtain the exact solutions in one of them analytically.
Conclusions: We have shown that different mechanisms of miRNA action lead to a variety of types of dynamical behavior of feed-forward loops. In particular, we found that the ability of feed-forward loop to dampen fluctuations introduced by transcription factor is the model and parameter dependent feature. We also discuss how our results could help a biologist to infer the mechanism of miRNA action.
Figures
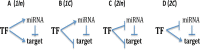
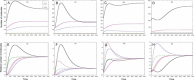
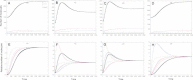
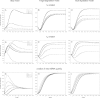
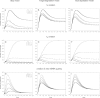
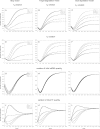
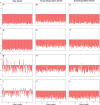
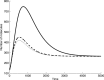
Similar articles
-
[The dynamics of feed-forward loop depends on regulator type in indirect pathway].Biofizika. 2015 Mar-Apr;60(2):213-24. Biofizika. 2015. PMID: 26016018 Russian.
-
Dynamic modeling of miRNA-mediated feed-forward loops.J Comput Biol. 2012 Feb;19(2):188-99. doi: 10.1089/cmb.2011.0274. J Comput Biol. 2012. PMID: 22300320
-
A Novel Five-Node Feed-Forward Loop Unravels miRNA-Gene-TF Regulatory Relationships in Ischemic Stroke.Mol Neurobiol. 2018 Nov;55(11):8251-8262. doi: 10.1007/s12035-018-0963-6. Epub 2018 Mar 9. Mol Neurobiol. 2018. PMID: 29524052
-
Modeling microRNA-transcription factor networks in cancer.Adv Exp Med Biol. 2013;774:149-67. doi: 10.1007/978-94-007-5590-1_9. Adv Exp Med Biol. 2013. PMID: 23377973 Review.
-
Mathematical modeling of microRNA-mediated mechanisms of translation repression.Adv Exp Med Biol. 2013;774:189-224. doi: 10.1007/978-94-007-5590-1_11. Adv Exp Med Biol. 2013. PMID: 23377975 Review.
Cited by
-
Understanding microRNA-mediated gene regulatory networks through mathematical modelling.Nucleic Acids Res. 2016 Jul 27;44(13):6019-35. doi: 10.1093/nar/gkw550. Epub 2016 Jun 17. Nucleic Acids Res. 2016. PMID: 27317695 Free PMC article.
-
M1 Microglia-derived Exosomes Promote Activation of Resting Microglia and Amplifies Proangiogenic Effects through Irf1/miR-155-5p/Socs1 Axis in the Retina.Int J Biol Sci. 2023 Mar 21;19(6):1791-1812. doi: 10.7150/ijbs.79784. eCollection 2023. Int J Biol Sci. 2023. PMID: 37063422 Free PMC article.
-
Modeling the Flowering Activation Motif during Vernalization in Legumes: A Case Study of M. trancatula.Life (Basel). 2023 Dec 23;14(1):26. doi: 10.3390/life14010026. Life (Basel). 2023. PMID: 38255642 Free PMC article.
-
Paternal programming of breast cancer risk in daughters in a rat model: opposing effects of animal- and plant-based high-fat diets.Breast Cancer Res. 2016 Jul 26;18(1):71. doi: 10.1186/s13058-016-0729-x. Breast Cancer Res. 2016. PMID: 27456846 Free PMC article.
-
Effect of Dynamic Interaction between microRNA and Transcription Factor on Gene Expression.Biomed Res Int. 2016;2016:2676282. doi: 10.1155/2016/2676282. Epub 2016 Nov 10. Biomed Res Int. 2016. PMID: 27957492 Free PMC article.
References
-
- Calin GA, Ferracin M, Cimmino A, Di Leva G, Shimizu M, Wojcik SE, lorio MV, Visone R, Sever NI, Fabbri M, Luliano R, Palumbo T, Pichiorri F, Roldo C, Garzon R, Sevignani C, Rassenti L, Alder H, Volinia S, Liu CG, Kipps TJ, Negrini M, Croce CM. A microRNA signature associated with prognosis and progression in chronic lymphocytic leukemia. N Engl J Med. 2005;353:1793–1801. doi: 10.1056/NEJMoa050995. - DOI - PubMed
Publication types
MeSH terms
Substances
LinkOut - more resources
Full Text Sources
Other Literature Sources
Research Materials