Mechanisms of activation of voltage-gated potassium channels
- PMID: 25558391
- PMCID: PMC4273088
Mechanisms of activation of voltage-gated potassium channels
Abstract
Voltage-gated potassium ion channels (Kv) play an important role in a variety of cellular processes, including the functioning of excitable cells, regulation of apoptosis, cell growth and differentiation, the release of neurotransmitters and hormones, maintenance of cardiac activity, etc. Failure in the functioning of Kv channels leads to severe genetic disorders and the development of tumors, including malignant ones. Understanding the mechanisms underlying Kv channels functioning is a key factor in determining the cause of the diseases associated with mutations in the channels, and in the search for new drugs. The mechanism of activation of the channels is a topic of ongoing debate, and a consensus on the issue has not yet been reached. This review discusses the key stages in studying the mechanisms of functioning of Kv channels and describes the basic models of their activation known to date.
Keywords: activation; modeling; potassium ion channels; structure.
Figures
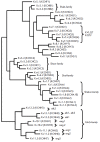
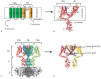
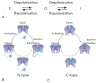
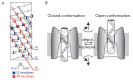
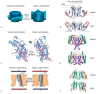
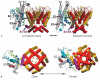
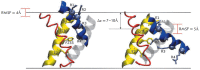
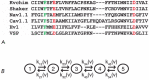
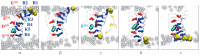
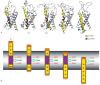
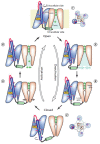
Similar articles
-
Differential effect of brief electrical stimulation on voltage-gated potassium channels.J Neurophysiol. 2017 May 1;117(5):2014-2024. doi: 10.1152/jn.00915.2016. Epub 2017 Feb 15. J Neurophysiol. 2017. PMID: 28202576 Free PMC article.
-
Molecular mechanisms of cardiac voltage-gated potassium channelopathies.Curr Pharm Des. 2006;12(28):3631-44. doi: 10.2174/138161206778522065. Curr Pharm Des. 2006. PMID: 17073664 Review.
-
A structural interpretation of voltage-gated potassium channel inactivation.Prog Biophys Mol Biol. 2006 Oct;92(2):185-208. doi: 10.1016/j.pbiomolbio.2005.10.001. Epub 2005 Nov 8. Prog Biophys Mol Biol. 2006. PMID: 16316679 Review.
-
Trafficking mechanisms underlying neuronal voltage-gated ion channel localization at the axon initial segment.Epilepsia. 2012 Dec;53 Suppl 9(Suppl 9):21-31. doi: 10.1111/epi.12032. Epilepsia. 2012. PMID: 23216576 Free PMC article. Review.
-
Mechanism of electromechanical coupling in voltage-gated potassium channels.Front Pharmacol. 2012 Sep 12;3:166. doi: 10.3389/fphar.2012.00166. eCollection 2012. Front Pharmacol. 2012. PMID: 22988442 Free PMC article.
Cited by
-
Voltage-gated ion channels mediate the electrotaxis of glioblastoma cells in a hybrid PMMA/PDMS microdevice.APL Bioeng. 2020 Jul 1;4(3):036102. doi: 10.1063/5.0004893. eCollection 2020 Sep. APL Bioeng. 2020. PMID: 32637857 Free PMC article.
-
Ion channels in sarcoma: pathophysiology and treatment options.Pflugers Arch. 2019 Sep;471(9):1163-1171. doi: 10.1007/s00424-019-02299-8. Epub 2019 Aug 3. Pflugers Arch. 2019. PMID: 31377822 Review.
-
Challenges and advances in atomistic simulations of potassium and sodium ion channel gating and permeation.J Physiol. 2019 Feb;597(3):679-698. doi: 10.1113/JP277088. Epub 2018 Dec 19. J Physiol. 2019. PMID: 30471114 Free PMC article. Review.
-
The A818-6 system as an in-vitro model for studying the role of the transportome in pancreatic cancer.BMC Cancer. 2020 Mar 30;20(1):264. doi: 10.1186/s12885-020-06773-w. BMC Cancer. 2020. PMID: 32228510 Free PMC article.
-
A Transcriptomic Survey of Ion Channel-Based Conotoxins in the Chinese Tubular Cone Snail (Conus betulinus).Mar Drugs. 2017 Jul 18;15(7):228. doi: 10.3390/md15070228. Mar Drugs. 2017. PMID: 28718820 Free PMC article.
References
-
- Yu F.H., Yarov-Yarovoy V., Gutman G.A., Catterall W.A.. Pharmacol. Rev. 2005;57:387–395. - PubMed
-
- Gutman G.A., Chandy K.G., Grissmer S., Lazdunski M., McKinnon D., Pardo L.A., Robertson G.A., Rudy B., Sanguinetti M.C., Stühmer W., Wang X.. Pharmacol. Rev. 2005;57:473–508. - PubMed
-
- Hoshi T., Zagotta W.N., Aldrich R.W.. Science. 1990;250:533–538. - PubMed
-
- Yellen G.. Nature. 2002;419:35–42. - PubMed
-
- Bosma M.M., Hille B.. Endocrinology. 1992;130:3411–3420. - PubMed
LinkOut - more resources
Full Text Sources