The SARS-coronavirus papain-like protease: structure, function and inhibition by designed antiviral compounds
- PMID: 25554382
- PMCID: PMC5896749
- DOI: 10.1016/j.antiviral.2014.12.015
The SARS-coronavirus papain-like protease: structure, function and inhibition by designed antiviral compounds
Abstract
Over 10 years have passed since the deadly human coronavirus that causes severe acute respiratory syndrome (SARS-CoV) emerged from the Guangdong Province of China. Despite the fact that the SARS-CoV pandemic infected over 8500 individuals, claimed over 800 lives and cost billions of dollars in economic loss worldwide, there still are no clinically approved antiviral drugs, vaccines or monoclonal antibody therapies to treat SARS-CoV infections. The recent emergence of the deadly human coronavirus that causes Middle East respiratory syndrome (MERS-CoV) is a sobering reminder that new and deadly coronaviruses can emerge at any time with the potential to become pandemics. Therefore, the continued development of therapeutic and prophylactic countermeasures to potentially deadly coronaviruses is warranted. The coronaviral proteases, papain-like protease (PLpro) and 3C-like protease (3CLpro), are attractive antiviral drug targets because they are essential for coronaviral replication. Although the primary function of PLpro and 3CLpro are to process the viral polyprotein in a coordinated manner, PLpro has the additional function of stripping ubiquitin and ISG15 from host-cell proteins to aid coronaviruses in their evasion of the host innate immune responses. Therefore, targeting PLpro with antiviral drugs may have an advantage in not only inhibiting viral replication but also inhibiting the dysregulation of signaling cascades in infected cells that may lead to cell death in surrounding, uninfected cells. This review provides an up-to-date discussion on the SARS-CoV papain-like protease including a brief overview of the SARS-CoV genome and replication followed by a more in-depth discussion on the structure and catalytic mechanism of SARS-CoV PLpro, the multiple cellular functions of SARS-CoV PLpro, the inhibition of SARS-CoV PLpro by small molecule inhibitors, and the prospect of inhibiting papain-like protease from other coronaviruses. This paper forms part of a series of invited articles in Antiviral Research on "From SARS to MERS: 10years of research on highly pathogenic human coronaviruses."
Keywords: 3C-like protease; MERS-CoV; Nsp3; Papain-like protease; SARS-CoV; Ubiquitin.
Copyright © 2014 Elsevier B.V. All rights reserved.
Figures
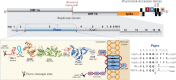
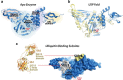
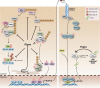
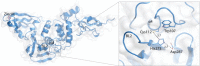
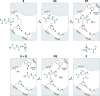
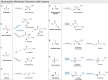
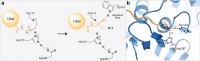
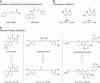
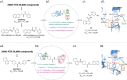
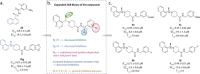
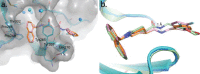
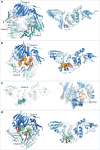
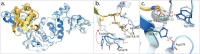
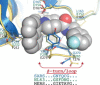
Similar articles
-
Structurally Guided Removal of DeISGylase Biochemical Activity from Papain-Like Protease Originating from Middle East Respiratory Syndrome Coronavirus.J Virol. 2017 Nov 14;91(23):e01067-17. doi: 10.1128/JVI.01067-17. Print 2017 Dec 1. J Virol. 2017. PMID: 28931677 Free PMC article.
-
Structural Basis for the Ubiquitin-Linkage Specificity and deISGylating activity of SARS-CoV papain-like protease.PLoS Pathog. 2014 May 22;10(5):e1004113. doi: 10.1371/journal.ppat.1004113. eCollection 2014 May. PLoS Pathog. 2014. PMID: 24854014 Free PMC article.
-
Structural and functional characterization of MERS coronavirus papain-like protease.J Biomed Sci. 2014 Jun 4;21(1):54. doi: 10.1186/1423-0127-21-54. J Biomed Sci. 2014. PMID: 24898546 Free PMC article.
-
Coronaviral PLpro proteases and the immunomodulatory roles of conjugated versus free Interferon Stimulated Gene product-15 (ISG15).Semin Cell Dev Biol. 2022 Dec;132:16-26. doi: 10.1016/j.semcdb.2022.06.005. Epub 2022 Jun 25. Semin Cell Dev Biol. 2022. PMID: 35764457 Free PMC article. Review.
-
Design and Evaluation of Anti-SARS-Coronavirus Agents Based on Molecular Interactions with the Viral Protease.Molecules. 2020 Aug 27;25(17):3920. doi: 10.3390/molecules25173920. Molecules. 2020. PMID: 32867349 Free PMC article. Review.
Cited by
-
Role of IFN and Complements System: Innate Immunity in SARS-CoV-2.J Inflamm Res. 2020 Sep 9;13:507-518. doi: 10.2147/JIR.S267280. eCollection 2020. J Inflamm Res. 2020. PMID: 32982366 Free PMC article. Review.
-
The role of kallikrein-kinin and renin-angiotensin systems in COVID-19 infection.Peptides. 2021 Jan;135:170428. doi: 10.1016/j.peptides.2020.170428. Epub 2020 Oct 14. Peptides. 2021. PMID: 33065209 Free PMC article. Review.
-
Class A G Protein-Coupled Receptor Antagonist Famotidine as a Therapeutic Alternative Against SARS-CoV2: An In Silico Analysis.Biomolecules. 2020 Jun 24;10(6):954. doi: 10.3390/biom10060954. Biomolecules. 2020. PMID: 32599963 Free PMC article.
-
The Discovery of Potential SARS-CoV-2 Natural Inhibitors among 4924 African Metabolites Targeting the Papain-like Protease: A Multi-Phase In Silico Approach.Metabolites. 2022 Nov 16;12(11):1122. doi: 10.3390/metabo12111122. Metabolites. 2022. PMID: 36422263 Free PMC article.
-
Assessment of proton-coupled conformational dynamics of SARS and MERS coronavirus papain-like proteases: Implication for designing broad-spectrum antiviral inhibitors.J Chem Phys. 2020 Sep 21;153(11):115101. doi: 10.1063/5.0020458. J Chem Phys. 2020. PMID: 32962355 Free PMC article.
References
-
- Baez-Santos Y.M. University of Illinois; Chicago: 2012. Insight into the Substrate Specificity and Inhibition of Human Coronavirus Papain-Like Proteases, Pharmacy. pp. 1–230.
-
- Baez-Santos Y.M., Barraza S.J., Wilson M.W., Agius M.P., Mielech A.M., Davis N.M., Baker S.C., Larsen S.D., Mesecar A.D. X-ray structural and biological evaluation of a series of potent and highly selective inhibitors of human coronavirus papain-like proteases. J. Med. Chem. 2014;57:2393–2412. - PMC - PubMed
-
- Barrett A.J., Rawlings N.D., Woessner J.F. Handbook of Proteolytic Enzymes. third ed. vol. 1. Elsevier Science; 2012. (Catalytic Mechanisms of Cysteine Peptidases). (chapter 404)
Publication types
MeSH terms
Substances
Grants and funding
LinkOut - more resources
Full Text Sources
Other Literature Sources
Miscellaneous