High-resolution HDX-MS reveals distinct mechanisms of RNA recognition and activation by RIG-I and MDA5
- PMID: 25539915
- PMCID: PMC4333383
- DOI: 10.1093/nar/gku1329
High-resolution HDX-MS reveals distinct mechanisms of RNA recognition and activation by RIG-I and MDA5
Abstract
RIG-I and MDA5 are the major intracellular immune receptors that recognize viral RNA species and undergo a series of conformational transitions leading to the activation of the interferon-mediated antiviral response. However, to date, full-length RLRs have resisted crystallographic efforts and a molecular description of their activation pathways remains hypothetical. Here we employ hydrogen/deuterium exchange coupled with mass spectrometry (HDX-MS) to probe the apo states of RIG-I and MDA5 and to dissect the molecular details with respect to distinct RNA species recognition, ATP binding and hydrolysis and CARDs activation. We show that human RIG-I maintains an auto-inhibited resting state owing to the intra-molecular HEL2i-CARD2 interactions while apo MDA5 lacks the analogous intra-molecular interactions and therefore adopts an extended conformation. Our work demonstrates that RIG-I binds and responds differently to short triphosphorylated RNA and long duplex RNA and that sequential addition of RNA and ATP triggers specific allosteric effects leading to RIG-I CARDs activation. We also present a high-resolution protein surface mapping technique that refines the cooperative oligomerization model of neighboring MDA5 molecules on long duplex RNA. Taken together, our data provide a high-resolution view of RLR activation in solution and offer new evidence for the molecular mechanism of RLR activation.
© The Author(s) 2014. Published by Oxford University Press on behalf of Nucleic Acids Research.
Figures
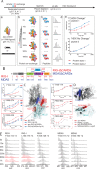
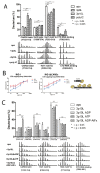
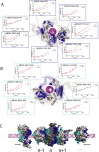
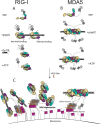
Similar articles
-
Kinetic discrimination of self/non-self RNA by the ATPase activity of RIG-I and MDA5.BMC Biol. 2015 Jul 28;13:54. doi: 10.1186/s12915-015-0166-9. BMC Biol. 2015. PMID: 26215161 Free PMC article.
-
Paramyxovirus V proteins disrupt the fold of the RNA sensor MDA5 to inhibit antiviral signaling.Science. 2013 Feb 8;339(6120):690-3. doi: 10.1126/science.1230949. Epub 2013 Jan 17. Science. 2013. PMID: 23328395
-
Structural basis of double-stranded RNA recognition by the RIG-I like receptor MDA5.Arch Biochem Biophys. 2009 Aug 1;488(1):23-33. doi: 10.1016/j.abb.2009.06.008. Epub 2009 Jun 14. Arch Biochem Biophys. 2009. PMID: 19531363
-
LGP2 synergy with MDA5 in RLR-mediated RNA recognition and antiviral signaling.Cytokine. 2015 Aug;74(2):198-206. doi: 10.1016/j.cyto.2015.02.010. Epub 2015 Mar 18. Cytokine. 2015. PMID: 25794939 Free PMC article. Review.
-
Parts, assembly and operation of the RIG-I family of motors.Curr Opin Struct Biol. 2014 Apr;25:25-33. doi: 10.1016/j.sbi.2013.11.011. Epub 2013 Dec 20. Curr Opin Struct Biol. 2014. PMID: 24878341 Free PMC article. Review.
Cited by
-
Proofreading mechanisms of the innate immune receptor RIG-I: distinguishing self and viral RNA.Biochem Soc Trans. 2024 Jun 26;52(3):1131-1148. doi: 10.1042/BST20230724. Biochem Soc Trans. 2024. PMID: 38884803 Free PMC article. Review.
-
Therapeutic Targeting of RIG-I and MDA5 Might Not Lead to the Same Rome.Trends Pharmacol Sci. 2019 Feb;40(2):116-127. doi: 10.1016/j.tips.2018.12.003. Epub 2018 Dec 31. Trends Pharmacol Sci. 2019. PMID: 30606502 Free PMC article. Review.
-
Unified mechanisms for self-RNA recognition by RIG-I Singleton-Merten syndrome variants.Elife. 2018 Jul 26;7:e38958. doi: 10.7554/eLife.38958. Elife. 2018. PMID: 30047865 Free PMC article.
-
The molecular mechanism of RIG-I activation and signaling.Immunol Rev. 2021 Nov;304(1):154-168. doi: 10.1111/imr.13022. Epub 2021 Sep 12. Immunol Rev. 2021. PMID: 34514601 Free PMC article. Review.
-
Short-interference RNAs: becoming medicines.EXCLI J. 2015 Jun 15;14:714-46. doi: 10.17179/excli2015-297. eCollection 2015. EXCLI J. 2015. PMID: 26648823 Free PMC article.
References
-
- Yoneyama M., Kikuchi M., Matsumoto K., Imaizumi T., Miyagishi M., Taira K., Foy E., Loo Y.M., Gale M., Jr, Akira S., et al. Shared and unique functions of the DExD/H-box helicases RIG-I, MDA5, and LGP2 in antiviral innate immunity. J. Immunol. 2005;175:2851–2858. - PubMed
-
- Yoneyama M., Kikuchi M., Natsukawa T., Shinobu N., Imaizumi T., Miyagishi M., Taira K., Akira S., Fujita T. The RNA helicase RIG-I has an essential function in double-stranded RNA-induced innate antiviral responses. Nat. Immunol. 2004;5:730–737. - PubMed
-
- Barbalat R., Ewald S.E., Mouchess M.L., Barton G.M. Nucleic acid recognition by the innate immune system. Annu. Rev. Immunol. 2011;29:185–214. - PubMed
Publication types
MeSH terms
Substances
LinkOut - more resources
Full Text Sources
Other Literature Sources
Research Materials