A Regulatory Network Involving β-Catenin, e-Cadherin, PI3k/Akt, and Slug Balances Self-Renewal and Differentiation of Human Pluripotent Stem Cells In Response to Wnt Signaling
- PMID: 25538040
- PMCID: PMC5297972
- DOI: 10.1002/stem.1944
A Regulatory Network Involving β-Catenin, e-Cadherin, PI3k/Akt, and Slug Balances Self-Renewal and Differentiation of Human Pluripotent Stem Cells In Response to Wnt Signaling
Abstract
The mechanisms underlying disparate roles of the canonical Wnt signaling pathway in maintaining self-renewal or inducing differentiation and lineage specification in embryonic stem cells (ESCs) are not clear. In this study, we provide the first demonstration that self-renewal versus differentiation of human ESCs (hESCs) in response to Wnt signaling is predominantly determined by a two-layer regulatory circuit involving β-catenin, E-cadherin, PI3K/Akt, and Slug in a time-dependent manner. Short-term upregulation of β-catenin does not lead to the activation of T-cell factor (TCF)-eGFP Wnt reporter in hESCs. Instead, it enhances E-cadherin expression on the cell membrane, thereby enhancing hESC self-renewal through E-cadherin-associated PI3K/Akt signaling. Conversely, long-term Wnt activation or loss of E-cadherin intracellular β-catenin binding domain induces TCF-eGFP activity and promotes hESC differentiation through β-catenin-induced upregulation of Slug. Enhanced expression of Slug leads to a further reduction of E-cadherin that serves as a β-catenin "sink" sequestering free cytoplasmic β-catenin. The formation of such a framework reinforces hESCs to switch from a state of temporal self-renewal associated with short-term Wnt/β-catenin activation to definitive differentiation. Stem Cells 2015;33:1419-1433.
Keywords: Differentiation; E-cadherin; Human embryonic stem cell; Self-renewal; Slug; Wnt; β-Catenin.
© 2015 AlphaMed Press.
Figures
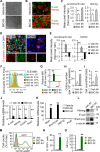
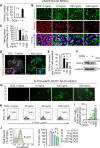
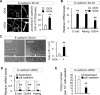
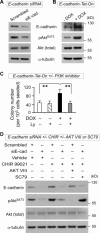
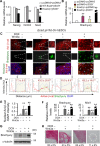
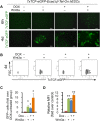
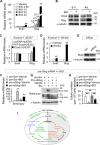
Similar articles
-
Progesterone Receptor Membrane Component 1 suppresses the p53 and Wnt/β-catenin pathways to promote human pluripotent stem cell self-renewal.Sci Rep. 2018 Feb 14;8(1):3048. doi: 10.1038/s41598-018-21322-z. Sci Rep. 2018. PMID: 29445107 Free PMC article.
-
β-Catenin in pluripotency: adhering to self-renewal or Wnting to differentiate?Int Rev Cell Mol Biol. 2014;312:53-78. doi: 10.1016/B978-0-12-800178-3.00002-6. Int Rev Cell Mol Biol. 2014. PMID: 25262238 Review.
-
Perturbed differentiation of murine embryonic stem cells upon Pelota deletion due to dysregulated FOXO1/β-catenin signaling.FEBS J. 2021 May;288(10):3317-3329. doi: 10.1111/febs.15643. Epub 2020 Dec 17. FEBS J. 2021. PMID: 33245852
-
Activation of Wnt/β-Catenin Signaling Pathway Enhances the Derivation of Buffalo (Bubalus bubalis) Embryonic Stem Cell-Like Cells.Cell Reprogram. 2020 Aug;22(4):217-225. doi: 10.1089/cell.2020.0027. Epub 2020 Jul 14. Cell Reprogram. 2020. PMID: 32673062
-
Maintaining embryonic stem cell pluripotency with Wnt signaling.Development. 2011 Oct;138(20):4341-50. doi: 10.1242/dev.066209. Epub 2011 Sep 8. Development. 2011. PMID: 21903672 Free PMC article. Review.
Cited by
-
DSG2 Is a Functional Cell Surface Marker for Identification and Isolation of Human Pluripotent Stem Cells.Stem Cell Reports. 2018 Jul 10;11(1):115-127. doi: 10.1016/j.stemcr.2018.05.009. Epub 2018 Jun 14. Stem Cell Reports. 2018. PMID: 29910125 Free PMC article.
-
Progesterone Receptor Membrane Component 1 suppresses the p53 and Wnt/β-catenin pathways to promote human pluripotent stem cell self-renewal.Sci Rep. 2018 Feb 14;8(1):3048. doi: 10.1038/s41598-018-21322-z. Sci Rep. 2018. PMID: 29445107 Free PMC article.
-
Effects of isoliquiritigenin on ovarian cancer cells.Onco Targets Ther. 2018 Mar 22;11:1633-1642. doi: 10.2147/OTT.S149295. eCollection 2018. Onco Targets Ther. 2018. PMID: 29606882 Free PMC article.
-
Gigantol Inhibits Epithelial to Mesenchymal Process in Human Lung Cancer Cells.Evid Based Complement Alternat Med. 2016;2016:4561674. doi: 10.1155/2016/4561674. Epub 2016 Aug 29. Evid Based Complement Alternat Med. 2016. PMID: 27651818 Free PMC article.
-
Glycans define the stemness of naïve and primed pluripotent stem cells.Glycoconj J. 2017 Dec;34(6):737-747. doi: 10.1007/s10719-016-9740-9. Epub 2016 Oct 28. Glycoconj J. 2017. PMID: 27796614 Review.
References
-
- Sato N, Meijer L, Skaltsounis L et al. Maintenance of pluripotency in human and mouse embryonic stem cells through activation of Wnt signaling by a pharmacological GSK‐3‐specific inhibitor. Nat Med 2004;10:55–63. - PubMed
-
- Singla DK, Schneider DJ, LeWinter MM et al. wnt3a but not wnt11 supports self‐renewal of embryonic stem cells. Biochem Biophys Res Commun 2006;345:789–795. - PubMed
-
- Miki T, Yasuda SY, Kahn M. Wnt/beta‐catenin signaling in embryonic stem cell self‐renewal and somatic cell reprogramming. Stem Cell Rev 2011;7:836–846. - PubMed
Publication types
MeSH terms
Substances
Grants and funding
LinkOut - more resources
Full Text Sources
Other Literature Sources
Molecular Biology Databases
Research Materials