Channelrhodopsin-assisted patching: in vivo recording of genetically and morphologically identified neurons throughout the brain
- PMID: 25533350
- PMCID: PMC4277210
- DOI: 10.1016/j.celrep.2014.11.042
Channelrhodopsin-assisted patching: in vivo recording of genetically and morphologically identified neurons throughout the brain
Abstract
Brain networks contain a large diversity of functionally distinct neuronal elements, each with unique properties, enabling computational capacities and supporting brain functions. Understanding their functional implications for behavior requires the precise identification of the cell types of a network and in vivo monitoring of their activity profiles. Here, we developed a channelrhodopsin-assisted patching method allowing the efficient in vivo targeted recording of neurons identified by their molecular, electrophysiological, and morphological features. The method has a high yield, does not require visual guidance, and thus can be applied at any depth in the brain. This approach overcomes limitations of present technologies. We validate this strategy with in vivo recordings of identified subtypes of GABAergic and glutamatergic neurons in deep cortical layers, subcortical cholinergic neurons, and neurons in the thalamic reticular nucleus in anesthetized and awake mice. We propose this method as an important complement to existing technologies to relate specific cell-type activity to brain circuitry, function, and behavior.
Copyright © 2014 The Authors. Published by Elsevier Inc. All rights reserved.
Figures
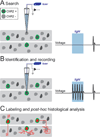
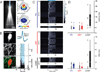
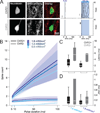
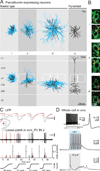
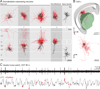
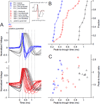
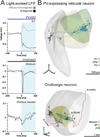
Similar articles
-
Acute brain slice methods for adult and aging animals: application of targeted patch clamp analysis and optogenetics.Methods Mol Biol. 2014;1183:221-42. doi: 10.1007/978-1-4939-1096-0_14. Methods Mol Biol. 2014. PMID: 25023312 Free PMC article.
-
Optogenetics to Interrogate Neuron-Glia Interactions in Pups and Adults.Methods Mol Biol. 2021;2191:135-149. doi: 10.1007/978-1-0716-0830-2_9. Methods Mol Biol. 2021. PMID: 32865743
-
Neostriatal GABAergic Interneurons Mediate Cholinergic Inhibition of Spiny Projection Neurons.J Neurosci. 2016 Sep 7;36(36):9505-11. doi: 10.1523/JNEUROSCI.0466-16.2016. J Neurosci. 2016. PMID: 27605623 Free PMC article.
-
In-vivo optogenetics and pharmacology in deep intracellular recordings.J Neurosci Methods. 2019 Sep 1;325:108324. doi: 10.1016/j.jneumeth.2019.108324. Epub 2019 Jul 6. J Neurosci Methods. 2019. PMID: 31288037 Review.
-
Whole-Cell Recording of Neuronal Membrane Potential during Behavior.Neuron. 2017 Sep 13;95(6):1266-1281. doi: 10.1016/j.neuron.2017.06.049. Neuron. 2017. PMID: 28910617 Review.
Cited by
-
Diversity and Function of Somatostatin-Expressing Interneurons in the Cerebral Cortex.Int J Mol Sci. 2019 Jun 17;20(12):2952. doi: 10.3390/ijms20122952. Int J Mol Sci. 2019. PMID: 31212931 Free PMC article. Review.
-
Automated microscope-independent fluorescence-guided micropipette.Biomed Opt Express. 2021 Jul 6;12(8):4689-4699. doi: 10.1364/BOE.431372. eCollection 2021 Aug 1. Biomed Opt Express. 2021. PMID: 34513218 Free PMC article.
-
Opto-juxtacellular interrogation of neural circuits in freely moving mice.Nat Protoc. 2023 Aug;18(8):2415-2440. doi: 10.1038/s41596-023-00842-7. Epub 2023 Jul 7. Nat Protoc. 2023. PMID: 37420087 Review.
-
Optogenetics Identification of a Neuronal Type with a Glass Optrode in Awake Mice.J Vis Exp. 2018 Jun 28;(136):57781. doi: 10.3791/57781. J Vis Exp. 2018. PMID: 30010633 Free PMC article.
-
Diversity and Connectivity of Layer 5 Somatostatin-Expressing Interneurons in the Mouse Barrel Cortex.J Neurosci. 2018 Feb 14;38(7):1622-1633. doi: 10.1523/JNEUROSCI.2415-17.2017. Epub 2018 Jan 11. J Neurosci. 2018. PMID: 29326172 Free PMC article.
References
-
- Burgalossi A, Herfst L, Von Heimendahl M, Forste H, Haskic K, Schmidt M, Brecht M. Microcircuits of functionally identified neurons in the rat medial entorhinal cortex. Neuron. 2011;70:773–786. - PubMed
Publication types
MeSH terms
Substances
Grants and funding
LinkOut - more resources
Full Text Sources
Other Literature Sources
Molecular Biology Databases