Tox: a multifunctional transcription factor and novel regulator of mammalian corticogenesis
- PMID: 25527292
- PMCID: PMC4388598
- DOI: 10.15252/embj.201490061
Tox: a multifunctional transcription factor and novel regulator of mammalian corticogenesis
Abstract
Major efforts are invested to characterize the factors controlling the proliferation of neural stem cells. During mammalian corticogenesis, our group has identified a small pool of genes that are transiently downregulated in the switch of neural stem cells to neurogenic division and reinduced in newborn neurons. Among these switch genes, we found Tox, a transcription factor with hitherto uncharacterized roles in the nervous system. Here, we investigated the role of Tox in corticogenesis by characterizing its expression at the tissue, cellular and temporal level. We found that Tox is regulated by calcineurin/Nfat signalling. Moreover, we combined DNA adenine methyltransferase identification (DamID) with deep sequencing to characterize the chromatin binding properties of Tox including its motif and downstream transcriptional targets including Sox2, Tbr2, Prox1 and other key factors. Finally, we manipulated Tox in the developing brain and validated its multiple roles in promoting neural stem cell proliferation and neurite outgrowth of newborn neurons. Our data provide a valuable resource to study the role of Tox in other tissues and highlight a novel key player in brain development.
Keywords: DamID sequencing; HMG‐box transcription factors; Tox; brain development; neural stem cells.
© 2014 The Authors.
Figures
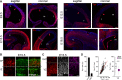
A-C Fluorescence pictures of WT (A and B) or Btg2RFP (C) mouse brains at E9.5, E12.5, E15.5 or E18.5 (as indicated) after immunohistochemistry for Tox (A-C; red), Tubb3 (B; green), Sox2 (C; white) or RFP (C; magenta) and DAPI counterstaining (A; blue).
D Quantification of Sox2+ or Btg2RFP+ cells within the Tox+ population (left; n = 3; error bars = SD) and immunoreactivity levels (au = arbitrary units) of each of the two makers relative to Tox represented as a linear correlation (middle; n = 79) or box plot (right; n ≥ 24), respectively. ***P < 0.001. Scale bars, 50 μm (A) or 40 μm (B).
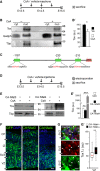
A-B′ Layout of CsA, or vehicle as control, administration during embryonic development (A) and Western blot analysis (B) of Tox levels in cytoplasmic (cyt) or nuclear (nuc) fractions of E15.5 brains. Quantifications (B′) were performed upon internal normalization for Gadph or Tbp and referred to treatment with vehicle. n = 3; error bars = SD; *P < 0.05.
C Map of Tox locus and Nfat-binding recognition sites (red) identified by MatInspector.
D-E′ Layout of CsA or vehicle administration and Western blot analysis as in (A–B′) upon in utero electroporation with CA-Nfat3 or CA-Nfat4 vectors at E13.5. Tox in extracts of E15.5 brains was quantified normalized to Tbp and referred to CsA treatment. n = 3; error bars = SD; ***P < 0.001.
F-G′ Fluorescent pictures of the mouse cortex upon electroporation with GFP, CA-Nfat3 or CA-Nfat4 (as indicated) followed by GFP (green) or Tox (G; red) immunolabelling and DAPI counterstaining (blue). Tox upregulation after CA-Nfat4 overexpression was assessed within nuclei of GFP+ cells (n = 30) in the VZ and represented as a box plot relative to GFP− cells (n = 30) used as internal control (G′). Tox ectopic expression within the SVZ is indicated (G; arrowheads). Scale bar, 50 μm (F) and 5 μm (G); **P < 0.01.
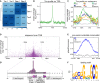
A Pearson's correlation analysis between Tox-Dam versus Dam reads of HEK-293T cells sampling the genome in windows of 10 kb.
B Meta-analysis of normalized Tox-Dam at the transcription start site (TSS) of Ensembl-annotated genes.
C Profile of normalized Tox-Dam reads on cell-specific enhancers (colours; categories ordered according to enrichment). Background (grey) was assessed based on the Tox signal at random genomic locations.
D Relationship between the fold-change of Tox peaks and their distance to the TSS. Note the enrichment in peaks at the boundary of ±15 kb from TSS and the correlation between their fold-change and proximity to the TSS.
D′ Distribution of Tox peaks within regions of loci (as indicated). The relative length of each genomic region was chosen to represent its average length across the human genome and circles distributed to represent the proportion of Tox peaks mapping on that given region (1 circle = 1%).
E PhastCons scores of 100 vertebrate genomes averaged for the Tox peak locations (blue) and for a matched genomic background (grey) showing higher conservation within Tox peaks (start-end).
F Putative 10-mer binding motif of Tox identified with gimmeMotifs.
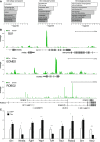
Functional enrichment scores for cellular compartment, molecular function and biological process (left to right).
Examples of Tox-Dam (green) or Dam (grey) peaks (superimposed in each panel) for Gli1, Eomes/Tbr2 and Robo2 (top to bottom) to represent factors involved in signalling, neurogenic commitment and neurite outgrowth, respectively.
qRT–PCRs of GFP+ FAC-sorted cells from the E15.5 mouse brain upon electroporation at E13.5 with control (white) or Tox (black) constructs. Primers (Supplementary Table S1) were selected to amplify multi-exon transcripts (indicated) to represent the classes of genes that would explain functional annotations in (A). n ≥ 3; error bars = SD; *P < 0.05; **P < 0.01; ***P < 0.001.
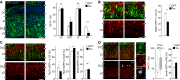
A-D Fluorescence pictures (left) and quantifications (right) of GFP+ (green) cells of the E15.5 lateral cortex positive for Btg2RFP (B), Tbr2 (C), Sox2 (D) (red) or p-Vim (D; white) upon electroporation with control or Tox (white or black bars, respectively) plasmids at E13.5. n ≥ 3; error bars = SD (except box plot in D, middle; n ≥ 50); ns = not significant; *P < 0.05; **P < 0.01; ***P < 0.001. Scale bars, 50 μm.
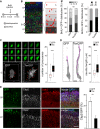
A-B′ Experimental layout (A), fluorescence pictures (B) and quantifications (B′) used to assess neuronal migration and output upon electroporation with GFP or Tox (B′; as indicated) plasmids at E13.5 followed by BrdU exposure and sacrifice as shown in (A). Neuronal migration was assessed by calculating the distribution of GFP+/BrdU+ cells within five equidistant bins of the IZ (B and B′; left), while output was quantified as the proportion of GFP+/BrdU+ in the IZ and CP relative to all GFP+ cells (B'; right). n = 2; error bars = SEM. Scale bar, 50 μm.
C, D 3D reconstruction (left) and quantification (right) of newborn (C) or polarized (D) neurons in the IZ or CP, respectively. Individual Z-stacks (0.1 μm optical thickness; green) and computer reconstructions (grey) were used to count cell protrusions (C; arrowheads) or neurite length (D; purple line) that were statistically evaluated (box plots; right, n ≥ 49; ***P < 0.001). Scale bars, 10 μm.
E Fluorescence pictures (left) of the E18.5 mouse cortex upon electroporation of GFP or Tox plasmids at E13.5 followed by immunohistochemistry for GFP (green), Ctip2 (white), Satb2 (red) and DAPI counterstaining (blue). Quantification of Ctip2+/GFP+ cells is shown in either condition (right). n = 3; **P < 0.01; error bars = SD. Scale bar, 50 μm.
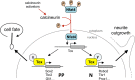
Comment in
-
In-TOX-icating neurogenesis.EMBO J. 2015 Apr 1;34(7):832-4. doi: 10.15252/embj.201591107. Epub 2015 Feb 17. EMBO J. 2015. PMID: 25691243 Free PMC article.
Similar articles
-
In-TOX-icating neurogenesis.EMBO J. 2015 Apr 1;34(7):832-4. doi: 10.15252/embj.201591107. Epub 2015 Feb 17. EMBO J. 2015. PMID: 25691243 Free PMC article.
-
Dynamic Expression of Sox2, Gata3, and Prox1 during Primary Auditory Neuron Development in the Mammalian Cochlea.PLoS One. 2017 Jan 24;12(1):e0170568. doi: 10.1371/journal.pone.0170568. eCollection 2017. PLoS One. 2017. PMID: 28118374 Free PMC article.
-
Identification of Tox chromatin binding properties and downstream targets by DamID-Seq.Genom Data. 2016 Feb 3;7:264-8. doi: 10.1016/j.gdata.2016.02.003. eCollection 2016 Mar. Genom Data. 2016. PMID: 26981424 Free PMC article.
-
NFAT: ubiquitous regulator of cell differentiation and adaptation.J Cell Biol. 2002 Mar 4;156(5):771-4. doi: 10.1083/jcb.200111073. Epub 2002 Mar 4. J Cell Biol. 2002. PMID: 11877454 Free PMC article. Review.
-
Post-transcriptional regulation in corticogenesis: how RNA-binding proteins help build the brain.Wiley Interdiscip Rev RNA. 2015 Sep-Oct;6(5):501-15. doi: 10.1002/wrna.1289. Epub 2015 Jun 18. Wiley Interdiscip Rev RNA. 2015. PMID: 26088328 Free PMC article. Review.
Cited by
-
Molecular Diversity of Midbrain Development in Mouse, Human, and Stem Cells.Cell. 2016 Oct 6;167(2):566-580.e19. doi: 10.1016/j.cell.2016.09.027. Cell. 2016. PMID: 27716510 Free PMC article.
-
Sequence and expression levels of circular RNAs in progenitor cell types during mouse corticogenesis.Life Sci Alliance. 2019 Mar 29;2(2):e201900354. doi: 10.26508/lsa.201900354. Print 2019 Apr. Life Sci Alliance. 2019. PMID: 30926618 Free PMC article.
-
Time-resolved transcriptomes reveal diverse B cell fate trajectories in the early response to Epstein-Barr virus infection.Cell Rep. 2022 Aug 30;40(9):111286. doi: 10.1016/j.celrep.2022.111286. Cell Rep. 2022. PMID: 36044865 Free PMC article.
-
The Emerging Roles of the Calcineurin-Nuclear Factor of Activated T-Lymphocytes Pathway in Nervous System Functions and Diseases.J Aging Res. 2016;2016:5081021. doi: 10.1155/2016/5081021. Epub 2016 Aug 15. J Aging Res. 2016. PMID: 27597899 Free PMC article. Review.
-
Identification and expression patterns of novel long non-coding RNAs in neural progenitors of the developing mammalian cortex.Neurogenesis (Austin). 2015 Apr 11;2(1):e995524. doi: 10.1080/23262133.2014.995524. eCollection 2015. Neurogenesis (Austin). 2015. PMID: 27504473 Free PMC article.
References
-
- Andersson R, Gebhard C, Miguel-Escalada I, Hoof I, Bornholdt J, Boyd M, Chen Y, Zhao X, Schmidl C, Suzuki T, Ntini E, Arner E, Valen E, Li K, Schwarzfischer L, Glatz D, Raithel J, Lilje B, Rapin N, Bagger FO, et al. An atlas of active enhancers across human cell types and tissues. Nature. 2014;507:455–461. - PMC - PubMed
Publication types
MeSH terms
Substances
LinkOut - more resources
Full Text Sources
Other Literature Sources
Molecular Biology Databases
Research Materials