Glucokinase activity in the arcuate nucleus regulates glucose intake
- PMID: 25485685
- PMCID: PMC4382228
- DOI: 10.1172/JCI77172
Glucokinase activity in the arcuate nucleus regulates glucose intake
Abstract
The brain relies on a constant supply of glucose, its primary fuel, for optimal function. A taste-independent mechanism within the CNS that promotes glucose delivery to the brain has been postulated to maintain glucose homeostasis; however, evidence for such a mechanism is lacking. Here, we determined that glucokinase activity within the hypothalamic arcuate nucleus is involved in regulation of dietary glucose intake. In fasted rats, glucokinase activity was specifically increased in the arcuate nucleus but not other regions of the hypothalamus. Moreover, pharmacologic and genetic activation of glucokinase in the arcuate nucleus of rodent models increased glucose ingestion, while decreased arcuate nucleus glucokinase activity reduced glucose intake. Pharmacologic targeting of potential downstream glucokinase effectors revealed that ATP-sensitive potassium channel and P/Q calcium channel activity are required for glucokinase-mediated glucose intake. Additionally, altered glucokinase activity affected release of the orexigenic neurotransmitter neuropeptide Y in response to glucose. Together, our results suggest that glucokinase activity in the arcuate nucleus specifically regulates glucose intake and that appetite for glucose is an important driver of overall food intake. Arcuate nucleus glucokinase activation may represent a CNS mechanism that underlies the oft-described phenomena of the "sweet tooth" and carbohydrate craving.
Figures
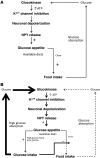
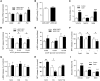
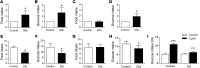
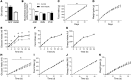
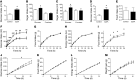
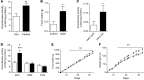
Similar articles
-
Arcuate nucleus glucokinase and dietary glucose intake.Oncotarget. 2015 Aug 21;6(24):19926-7. doi: 10.18632/oncotarget.5138. Oncotarget. 2015. PMID: 26343370 Free PMC article. No abstract available.
-
Hypothalamic arcuate nucleus glucokinase regulates insulin secretion and glucose homeostasis.Diabetes Obes Metab. 2018 Sep;20(9):2246-2254. doi: 10.1111/dom.13359. Epub 2018 Jun 12. Diabetes Obes Metab. 2018. PMID: 29748994 Free PMC article.
-
Pharmacological characterization and appetite suppressive properties of BMS-193885, a novel and selective neuropeptide Y(1) receptor antagonist.Eur J Pharmacol. 2008 Aug 20;590(1-3):224-32. doi: 10.1016/j.ejphar.2008.06.032. Epub 2008 Jun 12. Eur J Pharmacol. 2008. PMID: 18573246
-
Glucosensing neurons do more than just sense glucose.Int J Obes Relat Metab Disord. 2001 Dec;25 Suppl 5:S68-72. doi: 10.1038/sj.ijo.0801916. Int J Obes Relat Metab Disord. 2001. PMID: 11840219 Review.
-
Metabolic sensors: viewing glucosensing neurons from a broader perspective.Physiol Behav. 2002 Jul;76(3):397-401. doi: 10.1016/s0031-9384(02)00763-1. Physiol Behav. 2002. PMID: 12117576 Review.
Cited by
-
Sensing Glucose in the Central Melanocortin Circuits of Rainbow Trout: A Morphological Study.Front Endocrinol (Lausanne). 2019 Apr 18;10:254. doi: 10.3389/fendo.2019.00254. eCollection 2019. Front Endocrinol (Lausanne). 2019. PMID: 31057490 Free PMC article.
-
Glucose in the hypothalamic paraventricular nucleus regulates GLP-1 release.JCI Insight. 2020 Mar 31;5(8):e132760. doi: 10.1172/jci.insight.132760. JCI Insight. 2020. PMID: 32229720 Free PMC article.
-
A novel mammalian glucokinase exhibiting exclusive inorganic polyphosphate dependence in the cell nucleus.Biochem Biophys Rep. 2017 Sep 20;12:151-157. doi: 10.1016/j.bbrep.2017.09.004. eCollection 2017 Dec. Biochem Biophys Rep. 2017. PMID: 29090276 Free PMC article.
-
Recent advances in understanding hypothalamic control of defensive responses to hypoglycaemia.Curr Opin Endocr Metab Res. 2022 Jun;24:100353. doi: 10.1016/j.coemr.2022.100353. Curr Opin Endocr Metab Res. 2022. PMID: 39183767 Free PMC article. Review.
-
The shifting landscape of KATP channelopathies and the need for 'sharper' therapeutics.Future Med Chem. 2016 May;8(7):789-802. doi: 10.4155/fmc-2016-0005. Epub 2016 May 10. Future Med Chem. 2016. PMID: 27161588 Free PMC article. Review.
References
-
- Jacobs HL. Some physical, metabolic, and sensory components in the appetite for glucose. Am J Physiol. 1962;203:1043–1054. - PubMed
Publication types
MeSH terms
Substances
Grants and funding
- BB/E52708X/BB_/Biotechnology and Biological Sciences Research Council/United Kingdom
- 090792/Z/09/A/WT_/Wellcome Trust/United Kingdom
- BB/I00842X/1/BB_/Biotechnology and Biological Sciences Research Council/United Kingdom
- MC_U120027537/MRC_/Medical Research Council/United Kingdom
- BB/I00842X/BB_/Biotechnology and Biological Sciences Research Council/United Kingdom
LinkOut - more resources
Full Text Sources
Other Literature Sources
Molecular Biology Databases