Acceleration of α-synuclein aggregation by exosomes
- PMID: 25425650
- PMCID: PMC4317028
- DOI: 10.1074/jbc.M114.585703
Acceleration of α-synuclein aggregation by exosomes
Abstract
Exosomes are small vesicles released from cells into extracellular space. We have isolated exosomes from neuroblastoma cells and investigated their influence on the aggregation of α-synuclein, a protein associated with Parkinson disease pathology. Using cryo-transmission electron microscopy of exosomes, we found spherical unilamellar vesicles with a significant protein content, and Western blot analysis revealed that they contain, as expected, the proteins Flotillin-1 and Alix. Using thioflavin T fluorescence to monitor aggregation kinetics, we found that exosomes catalyze the process in a similar manner as a low concentration of preformed α-synuclein fibrils. The exosomes reduce the lag time indicating that they provide catalytic environments for nucleation. The catalytic effects of exosomes derived from naive cells and cells that overexpress α-synuclein do not differ. Vesicles prepared from extracted exosome lipids accelerate aggregation, suggesting that the lipids in exosomes are sufficient for the catalytic effect to arise. Using mass spectrometry, we found several phospholipid classes in the exosomes, including phosphatidylcholine, phosphatidylserine, phosphatidylethanolamine, phosphatidylinositol, and the gangliosides GM2 and GM3. Within each class, several species with different acyl chains were identified. We then prepared vesicles from corresponding pure lipids or defined mixtures, most of which were found to retard α-synuclein aggregation. As a striking exception, vesicles containing ganglioside lipids GM1 or GM3 accelerate the process. Understanding how α-synuclein interacts with biological membranes to promote neurological disease might lead to the identification of novel therapeutic targets.
Keywords: Amyloid; Exosome; Fibril; Fluorescence; Lipid; Mass Spectrometry (MS); Membrane; Parkinson Disease; Protein Aggregation; {alpha}-Synuclein.
© 2015 by The American Society for Biochemistry and Molecular Biology, Inc.
Figures
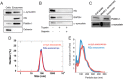
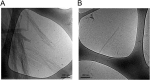
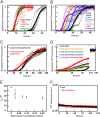
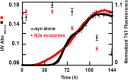
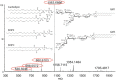
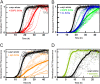
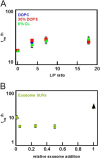
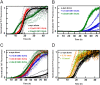
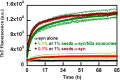
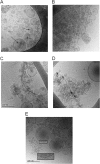
Similar articles
-
GM1 specifically interacts with alpha-synuclein and inhibits fibrillation.Biochemistry. 2007 Feb 20;46(7):1868-77. doi: 10.1021/bi061749a. Epub 2007 Jan 25. Biochemistry. 2007. PMID: 17253773
-
Microglial exosomes facilitate α-synuclein transmission in Parkinson's disease.Brain. 2020 May 1;143(5):1476-1497. doi: 10.1093/brain/awaa090. Brain. 2020. PMID: 32355963 Free PMC article.
-
Ganglioside GM3 stimulates lipid-protein co-assembly in α-synuclein amyloid formation.Biophys Chem. 2023 Feb;293:106934. doi: 10.1016/j.bpc.2022.106934. Epub 2022 Nov 21. Biophys Chem. 2023. PMID: 36493587
-
α-Synuclein-carrying extracellular vesicles in Parkinson's disease: deadly transmitters.Acta Neurol Belg. 2017 Mar;117(1):43-51. doi: 10.1007/s13760-016-0679-1. Epub 2016 Jul 29. Acta Neurol Belg. 2017. PMID: 27473175 Review.
-
The Role of Lipids Interacting with α-Synuclein in the Pathogenesis of Parkinson's Disease.J Parkinsons Dis. 2017;7(3):433-450. doi: 10.3233/JPD-171103. J Parkinsons Dis. 2017. PMID: 28671142 Review.
Cited by
-
Salivary total α-synuclein, oligomeric α-synuclein and SNCA variants in Parkinson's disease patients.Sci Rep. 2016 Jun 23;6:28143. doi: 10.1038/srep28143. Sci Rep. 2016. PMID: 27335051 Free PMC article.
-
Microglial Exosomes in Neurodegenerative Disease.Front Mol Neurosci. 2021 May 11;14:630808. doi: 10.3389/fnmol.2021.630808. eCollection 2021. Front Mol Neurosci. 2021. PMID: 34045943 Free PMC article. Review.
-
Selection of DNA aptamers that prevent the fibrillization of α-synuclein protein in cellular and mouse models.Mol Ther Nucleic Acids. 2024 Jun 15;35(3):102251. doi: 10.1016/j.omtn.2024.102251. eCollection 2024 Sep 10. Mol Ther Nucleic Acids. 2024. PMID: 39377064 Free PMC article.
-
Biomolecular condensates can both accelerate and suppress aggregation of α-synuclein.Sci Adv. 2022 Dec 2;8(48):eabq6495. doi: 10.1126/sciadv.abq6495. Epub 2022 Dec 2. Sci Adv. 2022. PMID: 36459561 Free PMC article.
-
Mutations associated with familial Parkinson's disease alter the initiation and amplification steps of α-synuclein aggregation.Proc Natl Acad Sci U S A. 2016 Sep 13;113(37):10328-33. doi: 10.1073/pnas.1604645113. Epub 2016 Aug 29. Proc Natl Acad Sci U S A. 2016. PMID: 27573854 Free PMC article.
References
-
- Bonini N. M., Giasson B. I. (2005) Snaring the function of α-synuclein. Cell 123, 359–361 - PubMed
-
- Cremades N., Cohen S. I., Deas E., Abramov A. Y., Chen A. Y., Orte A., Sandal M., Clarke R. W., Dunne P., Aprile F. A., Bertoncini C. W., Wood N. W., Knowles T. P., Dobson C. M., Klenerman D. (2012) Direct observation of the interconversion of normal and toxic forms of α-synuclein. Cell 149, 1048–1059 - PMC - PubMed
-
- Schapira A. H. (2009) Neurobiology and treatment of Parkinson's disease. Trends Pharmacol. Sci. 30, 41–47 - PubMed
Publication types
MeSH terms
Substances
LinkOut - more resources
Full Text Sources
Other Literature Sources
Research Materials
Miscellaneous