Identification of RISC-associated adenoviral microRNAs, a subset of their direct targets, and global changes in the targetome upon lytic adenovirus 5 infection
- PMID: 25410853
- PMCID: PMC4300742
- DOI: 10.1128/JVI.02336-14
Identification of RISC-associated adenoviral microRNAs, a subset of their direct targets, and global changes in the targetome upon lytic adenovirus 5 infection
Abstract
Adenoviruses encode a set of highly abundant microRNAs (mivaRNAs), which are generated by Dicer-mediated cleavage of the larger noncoding virus-associated RNAs (VA RNAs) I and II. We performed deep RNA sequencing to thoroughly investigate the relative abundance of individual single strands of mivaRNA isoforms in human A549 cells lytically infected with human adenovirus 5 (Ad5) at physiologically relevant multiplicities of infection (MOIs). In addition, we investigated their relative abundance in the endogenous RNA-induced silencing complexes (RISCs). The occupation of endogenous RISCs by mivaRNAs turned out to be pronounced but not as dominant as previously inferred from experiments with AGO2-overexpressing cells infected at high MOIs. In parallel, levels of RISC-incorporated mRNAs were investigated as well. Analysis of mRNAs enriched in RISCs in Ad5-infected cells revealed that only mRNAs with complementarity to the seed sequences of mivaRNAs derived from VA RNAI but not VA RNAII were overrepresented among them, indicating that only mivaRNAs derived from VA RNAI are likely to contribute substantially to the posttranscriptional downregulation of host gene expression. Furthermore, to generate a comprehensive picture of the entire transcriptome/targetome in lytically infected cells, we determined changes in cellular miRNA levels in both total RNA and RISC RNA as well, and bioinformatical analysis of mRNAs of total RNA/RISC fractions revealed a general, genome-wide trend toward detargeting of cellular mRNAs upon infection. Lastly, we identified the direct targets of both single strands of a VA RNAI-derived mivaRNA that constituted one of the two most abundant isoforms in RISCs of lytically infected A549 cells.
Importance: Viral and cellular miRNAs have been recognized as important players in virus-host interactions. This work provides the currently most comprehensive picture of the entire mRNA/miRNA transcriptome and of the complete RISC targetome during lytic adenovirus infection and thus represents the basis for a deeper understanding of the interplay between the virus and the cellular RNA interference machinery. Our data suggest that, at least in the model system that was employed, lytic infection by Ad5 is accompanied by a measurable global net detargeting effect on cellular mRNAs, and analysis of RISC-associated viral small RNAs revealed that the VA RNAs are the only source of virus-encoded miRNAs. Moreover, this work allows to assess the power of individual viral miRNAs to regulate cellular gene expression and provides a list of proven and putative direct targets of these miRNAs, which is of importance, given the fact that information about validated targets of adenovirus-encoded miRNAs is scarce.
Copyright © 2015, American Society for Microbiology. All Rights Reserved.
Figures
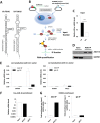
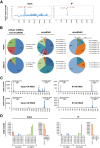
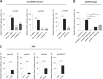
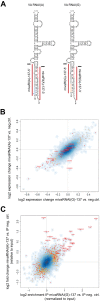
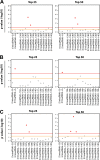
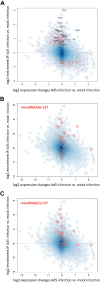
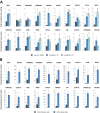
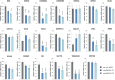
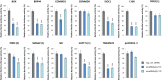
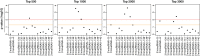
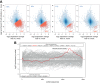
Similar articles
-
Small RNA sequence analysis of adenovirus VA RNA-derived miRNAs reveals an unexpected serotype-specific difference in structure and abundance.PLoS One. 2014 Aug 21;9(8):e105746. doi: 10.1371/journal.pone.0105746. eCollection 2014. PLoS One. 2014. PMID: 25144466 Free PMC article.
-
Characterization of Viral miRNAs during Adenovirus 14 Infection and Their Differential Expression in the Emergent Strain Adenovirus 14p1.Viruses. 2022 Apr 26;14(5):898. doi: 10.3390/v14050898. Viruses. 2022. PMID: 35632641 Free PMC article.
-
Adenovirus virus-associated RNAII-derived small RNAs are efficiently incorporated into the rna-induced silencing complex and associate with polyribosomes.J Virol. 2007 Oct;81(19):10540-9. doi: 10.1128/JVI.00885-07. Epub 2007 Jul 25. J Virol. 2007. PMID: 17652395 Free PMC article.
-
Adenovirus and miRNAs.Biochim Biophys Acta. 2011 Nov-Dec;1809(11-12):660-7. doi: 10.1016/j.bbagrm.2011.05.004. Epub 2011 May 17. Biochim Biophys Acta. 2011. PMID: 21621026 Free PMC article. Review.
-
[Development of Novel Genetically Engineered Adenoviruses Based on Functional Analyses of Adenovirus-encoded Small RNAs].Yakugaku Zasshi. 2016;136(11):1509-1515. doi: 10.1248/yakushi.16-00170. Yakugaku Zasshi. 2016. PMID: 27803482 Review. Japanese.
Cited by
-
The role of microRNAs in respiratory viral infection: friend or foe?Rev Med Virol. 2016 Nov;26(6):389-407. doi: 10.1002/rmv.1894. Epub 2016 Jul 4. Rev Med Virol. 2016. PMID: 27373545 Free PMC article. Review.
-
Viral miRNA regulation of host gene expression.Semin Cell Dev Biol. 2023 Sep 15;146:2-19. doi: 10.1016/j.semcdb.2022.11.007. Epub 2022 Nov 30. Semin Cell Dev Biol. 2023. PMID: 36463091 Free PMC article. Review.
-
Role of Host and Pathogen-Derived MicroRNAs in Immune Regulation During Infectious and Inflammatory Diseases.Front Immunol. 2020 Jan 24;10:3081. doi: 10.3389/fimmu.2019.03081. eCollection 2019. Front Immunol. 2020. PMID: 32038627 Free PMC article. Review.
-
Toward a Categorization of Virus-ncRNA Interactions in the World of RNA to Disentangle the Tiny Secrets of Dengue Virus.Viruses. 2024 May 18;16(5):804. doi: 10.3390/v16050804. Viruses. 2024. PMID: 38793685 Free PMC article. Review.
-
A MicroRNA Derived from Adenovirus Virus-Associated RNAII Promotes Virus Infection via Posttranscriptional Gene Silencing.J Virol. 2019 Jan 4;93(2):e01265-18. doi: 10.1128/JVI.01265-18. Print 2019 Jan 15. J Virol. 2019. PMID: 30355689 Free PMC article.
References
Publication types
MeSH terms
Substances
Grants and funding
LinkOut - more resources
Full Text Sources
Other Literature Sources
Molecular Biology Databases