Connexons and pannexons: newcomers in neurophysiology
- PMID: 25408635
- PMCID: PMC4219455
- DOI: 10.3389/fncel.2014.00348
Connexons and pannexons: newcomers in neurophysiology
Abstract
Connexin hemichannels are single membrane channels which have been traditionally thought to work in pairs to form gap junction channels across two opposing cells. In astrocytes, gap junction channels allow direct intercellular communication and greatly facilitate the transmission of signals. Recently, there has been growing evidence demonstrating that connexin hemichannels, as well as pannexin channels, on their own are open in various conditions. They allow bidirectional flow of ions and signaling molecules and act as release sites for transmitters like ATP and glutamate into the extracellular space. While much attention has focused on the function of connexin hemichannels and pannexons during pathological situations like epilepsy, inflammation, neurodegeneration or ischemia, their potential roles in physiology is often ignored. In order to fully understand the dynamic properties and roles of connexin hemichannels and pannexons in the brain, it is essential to decipher whether they also have some physiological functions and contribute to normal cerebral processes. Here, we present recent studies in the CNS suggesting emerging physiological functions of connexin hemichannels and pannexons in normal neuronal activity and behavior. We also discuss how these pioneer studies pave the way for future research to extend the physiological relevance of connexons and pannexons, and some fundamental issues yet to be addressed.
Keywords: astrocytes; connexins; hemichannels; learning and memory; neurons; pannexins; plasticity; synapses.
Figures
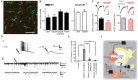
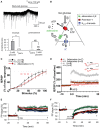
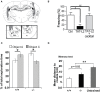
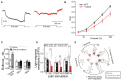
Similar articles
-
Connexin and pannexin hemichannels in inflammatory responses of glia and neurons.Brain Res. 2012 Dec 3;1487:3-15. doi: 10.1016/j.brainres.2012.08.042. Epub 2012 Sep 10. Brain Res. 2012. PMID: 22975435 Free PMC article. Review.
-
Synaptic Functions of Hemichannels and Pannexons: A Double-Edged Sword.Front Mol Neurosci. 2018 Dec 4;11:435. doi: 10.3389/fnmol.2018.00435. eCollection 2018. Front Mol Neurosci. 2018. PMID: 30564096 Free PMC article. Review.
-
Connexin and Pannexin-Based Channels in Oligodendrocytes: Implications in Brain Health and Disease.Front Cell Neurosci. 2019 Jan 29;13:3. doi: 10.3389/fncel.2019.00003. eCollection 2019. Front Cell Neurosci. 2019. PMID: 30760982 Free PMC article. Review.
-
Pannexin channels are not gap junction hemichannels.Channels (Austin). 2011 May-Jun;5(3):193-7. doi: 10.4161/chan.5.3.15765. Epub 2011 May 1. Channels (Austin). 2011. PMID: 21532340 Free PMC article. Review.
-
Gap junction channels and hemichannels in the CNS: regulation by signaling molecules.Neuropharmacology. 2013 Dec;75:567-82. doi: 10.1016/j.neuropharm.2013.02.020. Epub 2013 Mar 7. Neuropharmacology. 2013. PMID: 23499663 Review.
Cited by
-
P2X7 receptors and pannexin1 hemichannels shape presynaptic transmission.Purinergic Signal. 2024 Jun;20(3):223-236. doi: 10.1007/s11302-023-09965-8. Epub 2023 Sep 15. Purinergic Signal. 2024. PMID: 37713157 Free PMC article. Review.
-
Astrocytic and Oligodendrocytic P2X7 Receptors Determine Neuronal Functions in the CNS.Front Mol Neurosci. 2021 Feb 9;14:641570. doi: 10.3389/fnmol.2021.641570. eCollection 2021. Front Mol Neurosci. 2021. PMID: 33642994 Free PMC article.
-
Neurodegeneration in Multiple Sclerosis: The Role of Nrf2-Dependent Pathways.Antioxidants (Basel). 2022 Jun 10;11(6):1146. doi: 10.3390/antiox11061146. Antioxidants (Basel). 2022. PMID: 35740042 Free PMC article. Review.
-
Inhibition of Connexin43 Hemichannels Impairs Spatial Short-Term Memory without Affecting Spatial Working Memory.Front Cell Neurosci. 2016 Dec 20;10:288. doi: 10.3389/fncel.2016.00288. eCollection 2016. Front Cell Neurosci. 2016. PMID: 28066184 Free PMC article.
-
How Energy Metabolism Supports Cerebral Function: Insights from 13C Magnetic Resonance Studies In vivo.Front Neurosci. 2017 May 26;11:288. doi: 10.3389/fnins.2017.00288. eCollection 2017. Front Neurosci. 2017. PMID: 28603480 Free PMC article. Review.
References
-
- Anselmi F., Hernandez V. H., Crispino G., Seydel A., Ortolano S., Roper S. D., et al. . (2008). ATP release through connexin hemichannels and gap junction transfer of second messengers propagate Ca2+ signals across the inner ear. Proc. Natl. Acad. Sci. U.S.A. 105, 18770–18775. 10.1073/pnas.0800793105 - DOI - PMC - PubMed
Publication types
LinkOut - more resources
Full Text Sources
Other Literature Sources
Miscellaneous