NLRC5 interacts with RIG-I to induce a robust antiviral response against influenza virus infection
- PMID: 25404059
- PMCID: PMC11298762
- DOI: 10.1002/eji.201344412
NLRC5 interacts with RIG-I to induce a robust antiviral response against influenza virus infection
Abstract
The NLR protein, NLRC5 is an important regulator of MHC class I gene expression, however, the role of NLRC5 in other innate immune responses is less well defined. In the present study, we report that NLRC5 binds RIG-I and that this interaction is critical for robust antiviral responses against influenza virus. Overexpression of NLRC5 in the human lung epithelial cell line, A549, and normal human bronchial epithelial cells resulted in impaired replication of influenza virus A/Puerto Rico/8/34 virus (PR8) and enhanced IFN-β expression. Influenza virus leads to induction of IFN-β that drives RIG-I and NLRC5 expression in host cells. Our results suggest that NLRC5 extends and stabilizes influenza virus induced RIG-I expression and delays expression of the viral inhibitor protein NS1. We show that NS1 binds to NLRC5 to suppress its function. Interaction domain mapping revealed that NLRC5 interacts with RIG-I via its N-terminal death domain and that NLRC5 enhanced antiviral activity in an leucine-rich repeat domain independent manner. Taken together, our findings identify a novel role for NLRC5 in RIG-I-mediated antiviral host responses against influenza virus infection, distinguished from the role of NLRC5 in MHC class I gene regulation.
Keywords: Influenza; Interferon; NLRC5; NS1; RIG-I Antiviral.
© 2014 WILEY-VCH Verlag GmbH & Co. KGaA, Weinheim.
Conflict of interest statement
Figures
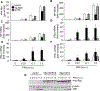
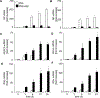
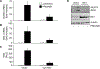
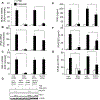
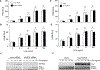
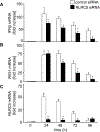
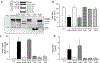
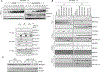
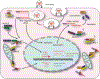
Similar articles
-
Respiratory Syncytial Virus Infection Upregulates NLRC5 and Major Histocompatibility Complex Class I Expression through RIG-I Induction in Airway Epithelial Cells.J Virol. 2015 Aug;89(15):7636-45. doi: 10.1128/JVI.00349-15. Epub 2015 May 13. J Virol. 2015. PMID: 25972545 Free PMC article.
-
Role of the Chaperone Protein 14-3-3ε in the Regulation of Influenza A Virus-Activated Beta Interferon.J Virol. 2021 Sep 27;95(20):e0023121. doi: 10.1128/JVI.00231-21. Epub 2021 Aug 11. J Virol. 2021. PMID: 34379499 Free PMC article.
-
IRF7 Is Required for the Second Phase Interferon Induction during Influenza Virus Infection in Human Lung Epithelia.Viruses. 2020 Mar 29;12(4):377. doi: 10.3390/v12040377. Viruses. 2020. PMID: 32235406 Free PMC article.
-
Respective roles of TLR, RIG-I and NLRP3 in influenza virus infection and immunity: impact on vaccine design.Expert Rev Vaccines. 2010 Nov;9(11):1315-24. doi: 10.1586/erv.10.118. Expert Rev Vaccines. 2010. PMID: 21087109 Review.
-
Innate Immune Sensing of Influenza A Virus.Viruses. 2020 Jul 14;12(7):755. doi: 10.3390/v12070755. Viruses. 2020. PMID: 32674269 Free PMC article. Review.
Cited by
-
Class I transactivator, NLRC5: a central player in the MHC class I pathway and cancer immune surveillance.Immunogenetics. 2019 Mar;71(3):273-282. doi: 10.1007/s00251-019-01106-z. Epub 2019 Jan 31. Immunogenetics. 2019. PMID: 30706093 Review.
-
Influenza virus NS1- C/EBPβ gene regulatory complex inhibits RIG-I transcription.Antiviral Res. 2020 Apr;176:104747. doi: 10.1016/j.antiviral.2020.104747. Epub 2020 Feb 21. Antiviral Res. 2020. PMID: 32092305 Free PMC article.
-
Critical role of RIG-I and MDA5 in early and late stages of Tulane virus infection.J Gen Virol. 2017 May;98(5):1016-1026. doi: 10.1099/jgv.0.000769. Epub 2017 May 22. J Gen Virol. 2017. PMID: 28530548 Free PMC article.
-
Alternative Pre-mRNA Splicing in Mammals and Teleost Fish: A Effective Strategy for the Regulation of Immune Responses Against Pathogen Infection.Int J Mol Sci. 2017 Jul 15;18(7):1530. doi: 10.3390/ijms18071530. Int J Mol Sci. 2017. PMID: 28714877 Free PMC article. Review.
-
Host Innate Antiviral Response to Influenza A Virus Infection: From Viral Sensing to Antagonism and Escape.Pathogens. 2024 Jul 3;13(7):561. doi: 10.3390/pathogens13070561. Pathogens. 2024. PMID: 39057788 Free PMC article. Review.
References
-
- Akira S, Uematsu S. and Takeuchi O, Pathogen recognition and innate immunity. Cell 2006. 124: 783–801. - PubMed
-
- Kawai T. and Akira S, TLR signaling. Cell Death Differ. 2006. 13: 816–825. - PubMed
-
- Ranjan P, Bowzard JB, Schwerzmann JW, Jeisy-Scott V, Fujita T. and Sambhara S, Cytoplasmic nucleic acid sensors in antiviral immunity. Trends Mol. Med 2009. 15: 359–368. - PubMed
-
- Takahasi K, Yoneyama M, Nishihori T, Hirai R, Kumeta H, Narita R, Gale M. et al., Nonself RNA-sensing mechanism of RIG-I helicase and activation of antiviral immune responses. Mol. Cell 2008. 29: 428–440. - PubMed
Publication types
MeSH terms
Substances
Grants and funding
LinkOut - more resources
Full Text Sources
Other Literature Sources
Medical
Research Materials