MUNC, a long noncoding RNA that facilitates the function of MyoD in skeletal myogenesis
- PMID: 25403490
- PMCID: PMC4285423
- DOI: 10.1128/MCB.01079-14
MUNC, a long noncoding RNA that facilitates the function of MyoD in skeletal myogenesis
Abstract
An in silico screen for myogenic long noncoding RNAs (lncRNAs) revealed nine lncRNAs that are upregulated more than 10-fold in myotubes versus levels in myoblasts. One of these lncRNAs, MyoD upstream noncoding (MUNC, also known as DRR(eRNA)), is encoded 5 kb upstream of the transcription start site of MyoD, a myogenic transcription factor gene. MUNC is specifically expressed in skeletal muscle and exists as in unspliced and spliced isoforms, and its 5' end overlaps with the cis-acting distal regulatory region (DRR) of MyoD. Small interfering RNA (siRNA) of MUNC reduced myoblast differentiation and specifically reduced the association of MyoD to the DRR enhancer and myogenin promoter but not to another MyoD-dependent enhancer. Stable overexpression of MUNC from a heterologous promoter increased endogenous MyoD, Myogenin, and Myh3 (myosin heavy chain, [MHC] gene) mRNAs but not the cognate proteins, suggesting that MUNC can act in trans to promote gene expression but that this activity does not require an induction of MyoD protein. MUNC also stimulates the transcription of other genes that are not recognized as MyoD-inducible genes. Knockdown of MUNC in vivo impaired murine muscle regeneration, implicating MUNC in primary satellite cell differentiation in the animal. We also discovered a human MUNC that is induced during differentiation of myoblasts and whose knockdown decreases differentiation, suggesting an evolutionarily conserved role of MUNC lncRNA in myogenesis. Although MUNC overlaps with the DRR enhancer, our results suggest that MUNC is not a classic cis-acting enhancer RNA (e-RNA) acting exclusively by stimulating the neighboring MyoD gene but more like a promyogenic lncRNA that acts directly or indirectly on multiple promoters to increase myogenic gene expression.
Copyright © 2015, American Society for Microbiology. All Rights Reserved.
Figures
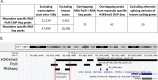
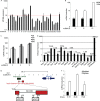
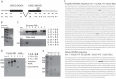
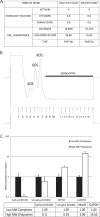
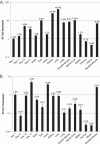
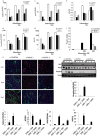
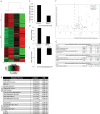
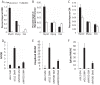
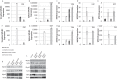
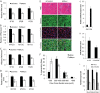
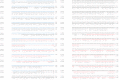
Similar articles
-
MUNC, an Enhancer RNA Upstream from the MYOD Gene, Induces a Subgroup of Myogenic Transcripts in trans Independently of MyoD.Mol Cell Biol. 2018 Sep 28;38(20):e00655-17. doi: 10.1128/MCB.00655-17. Print 2018 Oct 15. Mol Cell Biol. 2018. PMID: 30037979 Free PMC article.
-
S100B protein in myoblasts modulates myogenic differentiation via NF-kappaB-dependent inhibition of MyoD expression.J Cell Physiol. 2010 Apr;223(1):270-82. doi: 10.1002/jcp.22035. J Cell Physiol. 2010. PMID: 20069545
-
Tip60 regulates myoblast differentiation by enhancing the transcriptional activity of MyoD via their physical interactions.FEBS J. 2011 Nov;278(22):4394-404. doi: 10.1111/j.1742-4658.2011.08362.x. Epub 2011 Oct 17. FEBS J. 2011. PMID: 21936881
-
[Interactions of proliferation and differentiation signaling pathways in myogenesis].Postepy Hig Med Dosw (Online). 2014 May 8;68:516-26. doi: 10.5604/17322693.1101617. Postepy Hig Med Dosw (Online). 2014. PMID: 24864103 Review. Polish.
-
Regulation of Skeletal Myoblast Differentiation by Drebrin.Adv Exp Med Biol. 2017;1006:361-373. doi: 10.1007/978-4-431-56550-5_22. Adv Exp Med Biol. 2017. PMID: 28865032 Review.
Cited by
-
MEG3 Promotes Differentiation of Porcine Satellite Cells by Sponging miR-423-5p to Relieve Inhibiting Effect on SRF.Cells. 2020 Feb 15;9(2):449. doi: 10.3390/cells9020449. Cells. 2020. PMID: 32075310 Free PMC article.
-
Epigenetics and Shared Molecular Processes in the Regeneration of Complex Structures.Stem Cells Int. 2016;2016:6947395. doi: 10.1155/2016/6947395. Epub 2015 Nov 22. Stem Cells Int. 2016. PMID: 26681954 Free PMC article. Review.
-
Hormonal vitamin D up-regulates tissue-specific PD-L1 and PD-L2 surface glycoprotein expression in humans but not mice.J Biol Chem. 2017 Dec 15;292(50):20657-20668. doi: 10.1074/jbc.M117.793885. Epub 2017 Oct 23. J Biol Chem. 2017. PMID: 29061851 Free PMC article.
-
MUNC, an Enhancer RNA Upstream from the MYOD Gene, Induces a Subgroup of Myogenic Transcripts in trans Independently of MyoD.Mol Cell Biol. 2018 Sep 28;38(20):e00655-17. doi: 10.1128/MCB.00655-17. Print 2018 Oct 15. Mol Cell Biol. 2018. PMID: 30037979 Free PMC article.
-
An Emerging Role for Epigenetics in Cerebral Palsy.J Pers Med. 2021 Nov 12;11(11):1187. doi: 10.3390/jpm11111187. J Pers Med. 2021. PMID: 34834539 Free PMC article. Review.
References
-
- Clark MB, Amaral PP, Schlesinger FJ, Dinger ME, Taft RJ, Rinn JL, Ponting CP, Stadler PF, Morris KV, Morillon A, Rozowsky JS, Gerstein MB, Wahlestedt C, Hayashizaki Y, Carninci P, Gingeras TR, Mattick JS. 2011. The reality of pervasive transcription. PLoS Biol 9:e1000625. doi:10.1371/journal.pbio.1000625. - DOI - PMC - PubMed
-
- Derrien T, Johnson R, Bussotti G, Tanzer A, Djebali S, Tilgner H, Guernec G, Martin D, Merkel A, Knowles DG, Lagarde J, Veeravalli L, Ruan X, Ruan Y, Lassmann T, Carninci P, Brown JB, Lipovich L, Gonzalez JM, Thomas M, Davis CA, Shiekhattar R, Gingeras TR, Hubbard TJ, Notredame C, Harrow J, Guigó R. 2012. The GENCODE v7 catalog of human long noncoding RNAs: analysis of their gene structure, evolution, and expression. Genome Res 22:1775–1789. doi:10.1101/gr.132159.111. - DOI - PMC - PubMed
-
- Guttman M, Garber M, Levin JZ, Donaghey J, Robinson J, Adiconis X, Fan L, Koziol MJ, Gnirke A, Nusbaum C, Rinn JL, Lander ES, Regev A. 2010. Ab initio reconstruction of cell type-specific transcriptomes in mouse reveals the conserved multi-exonic structure of lincRNAs. Nat Biotechnol 28:503–510. doi:10.1038/nbt.1633. - DOI - PMC - PubMed
Publication types
MeSH terms
Substances
Grants and funding
LinkOut - more resources
Full Text Sources
Other Literature Sources
Molecular Biology Databases
Research Materials
Miscellaneous