Chromosome rearrangements via template switching between diverged repeated sequences
- PMID: 25367035
- PMCID: PMC4215184
- DOI: 10.1101/gad.250258.114
Chromosome rearrangements via template switching between diverged repeated sequences
Abstract
Recent high-resolution genome analyses of cancer and other diseases have revealed the occurrence of microhomology-mediated chromosome rearrangements and copy number changes. Although some of these rearrangements appear to involve nonhomologous end-joining, many must have involved mechanisms requiring new DNA synthesis. Models such as microhomology-mediated break-induced replication (MM-BIR) have been invoked to explain these rearrangements. We examined BIR and template switching between highly diverged sequences in Saccharomyces cerevisiae, induced during repair of a site-specific double-strand break (DSB). Our data show that such template switches are robust mechanisms that give rise to complex rearrangements. Template switches between highly divergent sequences appear to be mechanistically distinct from the initial strand invasions that establish BIR. In particular, such jumps are less constrained by sequence divergence and exhibit a different pattern of microhomology junctions. BIR traversing repeated DNA sequences frequently results in complex translocations analogous to those seen in mammalian cells. These results suggest that template switching among repeated genes is a potent driver of genome instability and evolution.
Keywords: break-induced replication; chromosome rearrangements; chromothripsis; template switching.
© 2014 Anand et al.; Published by Cold Spring Harbor Laboratory Press.
Figures
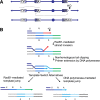
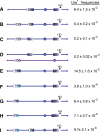
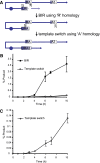
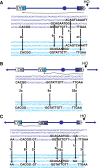
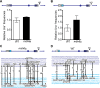
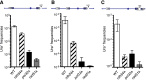
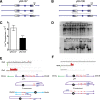
Similar articles
-
Template switching during break-induced replication.Nature. 2007 May 3;447(7140):102-5. doi: 10.1038/nature05723. Epub 2007 Apr 4. Nature. 2007. PMID: 17410126
-
Complex chromosomal rearrangements mediated by break-induced replication involve structure-selective endonucleases.PLoS Genet. 2012 Sep;8(9):e1002979. doi: 10.1371/journal.pgen.1002979. Epub 2012 Sep 27. PLoS Genet. 2012. PMID: 23071463 Free PMC article.
-
Break-induced replication links microsatellite expansion to complex genome rearrangements.Bioessays. 2017 Aug;39(8):10.1002/bies.201700025. doi: 10.1002/bies.201700025. Epub 2017 Jun 16. Bioessays. 2017. PMID: 28621832 Free PMC article. Review.
-
RAD51-dependent break-induced replication differs in kinetics and checkpoint responses from RAD51-mediated gene conversion.Mol Cell Biol. 2005 Feb;25(3):933-44. doi: 10.1128/MCB.25.3.933-944.2005. Mol Cell Biol. 2005. PMID: 15657422 Free PMC article.
-
Genomic rearrangements induced by unscheduled DNA double strand breaks in somatic mammalian cells.FEBS J. 2017 Aug;284(15):2324-2344. doi: 10.1111/febs.14053. Epub 2017 Mar 22. FEBS J. 2017. PMID: 28244221 Review.
Cited by
-
Repair of DNA Breaks by Break-Induced Replication.Annu Rev Biochem. 2021 Jun 20;90:165-191. doi: 10.1146/annurev-biochem-081420-095551. Epub 2021 Apr 1. Annu Rev Biochem. 2021. PMID: 33792375 Free PMC article. Review.
-
A Rad51-independent pathway promotes single-strand template repair in gene editing.PLoS Genet. 2020 Oct 15;16(10):e1008689. doi: 10.1371/journal.pgen.1008689. eCollection 2020 Oct. PLoS Genet. 2020. PMID: 33057349 Free PMC article.
-
Alternative Lengthening of Telomeres in Yeast: Old Questions and New Approaches.Biomolecules. 2024 Jan 16;14(1):113. doi: 10.3390/biom14010113. Biomolecules. 2024. PMID: 38254712 Free PMC article. Review.
-
Translesion Polymerases Drive Microhomology-Mediated Break-Induced Replication Leading to Complex Chromosomal Rearrangements.Mol Cell. 2015 Dec 17;60(6):860-72. doi: 10.1016/j.molcel.2015.10.041. Epub 2015 Dec 6. Mol Cell. 2015. PMID: 26669261 Free PMC article.
-
Deciphering the Code of the Cancer Genome: Mechanisms of Chromosome Rearrangement.Trends Cancer. 2015 Dec 1;1(4):217-230. doi: 10.1016/j.trecan.2015.10.007. Trends Cancer. 2015. PMID: 26726318 Free PMC article.
References
-
- Aguilera A, Gomez-Gonzalez B. 2008. Genome instability: a mechanistic view of its causes and consequences. Nat Rev Genet 9: 204–217 - PubMed
-
- Chiang C, Jacobsen JC, Ernst C, Hanscom C, Heilbut A, Blumenthal I, Mills RE, Kirby A, Lindgren AM, Rudiger SRet al. . 2012. Complex reorganization and predominant non-homologous repair following chromosomal breakage in karyotypically balanced germline rearrangements and transgenic integration. Nat Genet 44: 390–397, S391 - PMC - PubMed
Publication types
MeSH terms
Substances
Grants and funding
LinkOut - more resources
Full Text Sources
Other Literature Sources
Molecular Biology Databases