Signalling dynamics in the spindle checkpoint response
- PMID: 25303117
- PMCID: PMC4283840
- DOI: 10.1038/nrm3888
Signalling dynamics in the spindle checkpoint response
Abstract
The spindle checkpoint ensures proper chromosome segregation during cell division. Unravelling checkpoint signalling has been a long-standing challenge owing to the complexity of the structures and forces that regulate chromosome segregation. New reports have now substantially advanced our understanding of checkpoint signalling mechanisms at the kinetochore, the structure that connects microtubules and chromatin. In contrast to the traditional view of a binary checkpoint response - either completely on or off - new findings indicate that the checkpoint response strength is variable. This revised perspective provides insight into how checkpoint bypass can lead to aneuploidy and informs strategies to exploit these errors for cancer treatments.
Figures
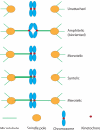
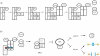
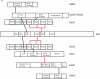
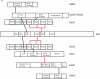
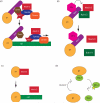
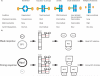
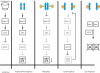
Similar articles
-
How the SAC gets the axe: Integrating kinetochore microtubule attachments with spindle assembly checkpoint signaling.Bioarchitecture. 2015;5(1-2):1-12. doi: 10.1080/19490992.2015.1090669. Epub 2015 Oct 2. Bioarchitecture. 2015. PMID: 26430805 Free PMC article. Review.
-
Microtubule attachment and spindle assembly checkpoint signalling at the kinetochore.Nat Rev Mol Cell Biol. 2013 Jan;14(1):25-37. doi: 10.1038/nrm3494. Nat Rev Mol Cell Biol. 2013. PMID: 23258294 Free PMC article. Review.
-
Attachment and tension in the spindle assembly checkpoint.J Cell Sci. 2002 Sep 15;115(Pt 18):3547-55. doi: 10.1242/jcs.00029. J Cell Sci. 2002. PMID: 12186941 Review.
-
Recent Progress on the Localization of PLK1 to the Kinetochore and Its Role in Mitosis.Int J Mol Sci. 2022 May 8;23(9):5252. doi: 10.3390/ijms23095252. Int J Mol Sci. 2022. PMID: 35563642 Free PMC article. Review.
-
Bub1 and BubR1: at the interface between chromosome attachment and the spindle checkpoint.Mol Cell Biol. 2011 Aug;31(15):3085-93. doi: 10.1128/MCB.05326-11. Epub 2011 May 31. Mol Cell Biol. 2011. PMID: 21628528 Free PMC article. Review.
Cited by
-
Short- and long-term effects of chromosome mis-segregation and aneuploidy.Nat Rev Mol Cell Biol. 2015 Aug;16(8):473-85. doi: 10.1038/nrm4025. Nat Rev Mol Cell Biol. 2015. PMID: 26204159 Review.
-
The Phosphatase Dusp7 Drives Meiotic Resumption and Chromosome Alignment in Mouse Oocytes.Cell Rep. 2016 Oct 25;17(5):1426-1437. doi: 10.1016/j.celrep.2016.10.007. Cell Rep. 2016. PMID: 27783954 Free PMC article.
-
The Error-Prone Kinetochore-Microtubule Attachments During Meiosis I in Vitrified Oocytes.Front Cell Dev Biol. 2020 Jul 9;8:621. doi: 10.3389/fcell.2020.00621. eCollection 2020. Front Cell Dev Biol. 2020. PMID: 32733898 Free PMC article.
-
Role of ubiquitin-protein ligase UBR5 in the disassembly of mitotic checkpoint complexes.Proc Natl Acad Sci U S A. 2022 Mar 1;119(9):e2121478119. doi: 10.1073/pnas.2121478119. Proc Natl Acad Sci U S A. 2022. PMID: 35217622 Free PMC article.
-
Deletion of APC7 or APC16 Allows Proliferation of Human Cells without the Spindle Assembly Checkpoint.Cell Rep. 2018 Nov 27;25(9):2317-2328.e5. doi: 10.1016/j.celrep.2018.10.104. Cell Rep. 2018. PMID: 30485802 Free PMC article.
References
Publication types
MeSH terms
Substances
Grants and funding
LinkOut - more resources
Full Text Sources
Other Literature Sources