Use of Caenorhabditis elegans as a model to study Alzheimer's disease and other neurodegenerative diseases
- PMID: 25250042
- PMCID: PMC4155875
- DOI: 10.3389/fgene.2014.00279
Use of Caenorhabditis elegans as a model to study Alzheimer's disease and other neurodegenerative diseases
Abstract
Advances in research and technology has increased our quality of life, allowed us to combat diseases, and achieve increased longevity. Unfortunately, increased longevity is accompanied by a rise in the incidences of age-related diseases such as Alzheimer's disease (AD). AD is the sixth leading cause of death, and one of the leading causes of dementia amongst the aged population in the USA. It is a progressive neurodegenerative disorder, characterized by the prevalence of extracellular Aβ plaques and intracellular neurofibrillary tangles, derived from the proteolysis of the amyloid precursor protein (APP) and the hyperphosphorylation of microtubule-associated protein tau, respectively. Despite years of extensive research, the molecular mechanisms that underlie the pathology of AD remain unclear. Model organisms, such as the nematode, Caenorhabditis elegans, present a complementary approach to addressing these questions. C. elegans has many advantages as a model system to study AD and other neurodegenerative diseases. Like their mammalian counterparts, they have complex biochemical pathways, most of which are conserved. Genes in which mutations are correlated with AD have counterparts in C. elegans, including an APP-related gene, apl-1, a tau homolog, ptl-1, and presenilin homologs, such as sel-12 and hop-1. Since the neuronal connectivity in C. elegans has already been established, C. elegans is also advantageous in modeling learning and memory impairments seen during AD. This article addresses the insights C. elegans provide in studying AD and other neurodegenerative diseases. Additionally, we explore the advantages and drawbacks associated with using this model.
Keywords: ALS; Alzheimer’s disease; C. elegans; Parkinson disease; apl-1; model systems.
Figures
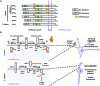
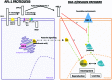
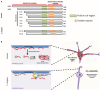
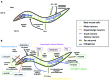
Similar articles
-
Understanding the molecular basis of Alzheimer's disease using a Caenorhabditis elegans model system.Brain Struct Funct. 2010 Mar;214(2-3):263-83. doi: 10.1007/s00429-009-0235-3. Epub 2009 Dec 11. Brain Struct Funct. 2010. PMID: 20012092 Free PMC article. Review.
-
Human amyloid β peptide and tau co-expression impairs behavior and causes specific gene expression changes in Caenorhabditis elegans.Neurobiol Dis. 2018 Jan;109(Pt A):88-101. doi: 10.1016/j.nbd.2017.10.003. Epub 2017 Oct 2. Neurobiol Dis. 2018. PMID: 28982592
-
Caenorhabditis elegans as an experimental tool for the study of complex neurological diseases: Parkinson's disease, Alzheimer's disease and autism spectrum disorder.Invert Neurosci. 2011 Dec;11(2):73-83. doi: 10.1007/s10158-011-0126-1. Epub 2011 Nov 8. Invert Neurosci. 2011. PMID: 22068627 Review.
-
Alzheimer's disease.Subcell Biochem. 2012;65:329-52. doi: 10.1007/978-94-007-5416-4_14. Subcell Biochem. 2012. PMID: 23225010 Review.
-
Therapeutic potentials of plant iridoids in Alzheimer's and Parkinson's diseases: A review.Eur J Med Chem. 2019 May 1;169:185-199. doi: 10.1016/j.ejmech.2019.03.009. Epub 2019 Mar 8. Eur J Med Chem. 2019. PMID: 30877973 Review.
Cited by
-
Meta-analysis of Genetic Modifiers Reveals Candidate Dysregulated Pathways in Amyotrophic Lateral Sclerosis.Neuroscience. 2019 Jan 1;396:A3-A20. doi: 10.1016/j.neuroscience.2018.10.033. Neuroscience. 2019. PMID: 30594291 Free PMC article.
-
Virus-Induced Membrane Fusion in Neurodegenerative Disorders.Front Cell Infect Microbiol. 2022 Mar 24;12:845580. doi: 10.3389/fcimb.2022.845580. eCollection 2022. Front Cell Infect Microbiol. 2022. PMID: 35531328 Free PMC article. Review.
-
Promising Molecular Targets in Pharmacological Therapy for Neuronal Damage in Brain Injury.Antioxidants (Basel). 2023 Jan 3;12(1):118. doi: 10.3390/antiox12010118. Antioxidants (Basel). 2023. PMID: 36670980 Free PMC article. Review.
-
αS1-Casein-Loaded Proteo-liposomes as Potential Inhibitors in Amyloid Fibrillogenesis: In Vivo Effects on a C. elegans Model of Alzheimer's Disease.ACS Chem Neurosci. 2023 Nov 1;14(21):3894-3904. doi: 10.1021/acschemneuro.3c00239. Epub 2023 Oct 17. ACS Chem Neurosci. 2023. PMID: 37847529 Free PMC article.
-
Generation and characterization of a tractable C. elegans model of tauopathy.Geroscience. 2021 Oct;43(5):2621-2631. doi: 10.1007/s11357-021-00436-9. Epub 2021 Sep 18. Geroscience. 2021. PMID: 34536202 Free PMC article.
References
-
- Aizawa H., Emori Y., Murofushi H., Kawasaki H., Sakai H., Suzuki K. (1990). Molecular cloning of a ubiquitously distributed microtubule-associated protein with Mr 190,000. J. Biol. Chem. 265 13849–13855 - PubMed
-
- Alonso A. D., Grundke-Iqbal I., Barra H. S., Iqbal K. (1997). Abnormal phosphorylation of tau and the mechanism of Alzheimer neurofibrillary degeneration: sequestration of microtubule-associated proteins 1 and 2 and the disassembly of microtubules by the abnormal tau. Proc. Natl. Acad. Sci. U.S.A. 94 298–303 10.1073/pnas.94.1.298 - DOI - PMC - PubMed
Publication types
Grants and funding
LinkOut - more resources
Full Text Sources
Other Literature Sources
Miscellaneous