Current state of laser synthesis of metal and alloy nanoparticles as ligand-free reference materials for nano-toxicological assays
- PMID: 25247135
- PMCID: PMC4168911
- DOI: 10.3762/bjnano.5.165
Current state of laser synthesis of metal and alloy nanoparticles as ligand-free reference materials for nano-toxicological assays
Abstract
Due to the abundance of nanomaterials in medical devices and everyday products, toxicological effects related to nanoparticles released from these materials, e.g., by mechanical wear, are a growing matter of concern. Unfortunately, appropriate nanoparticles required for systematic toxicological evaluation of these materials are still lacking. Here, the ubiquitous presence of surface ligands, remaining from chemical synthesis are a major drawback as these organic residues may cause cross-contaminations in toxicological studies. Nanoparticles synthesized by pulsed laser ablation in liquid are a promising alternative as this synthesis route provides totally ligand-free nanoparticles. The first part of this article reviews recent methods that allow the size control of laser-fabricated nanoparticles, focusing on laser post irradiation, delayed bioconjugation and in situ size quenching by low salinity electrolytes. Subsequent or parallel applications of these methods enable precise tuning of the particle diameters in a regime from 4-400 nm without utilization of any artificial surface ligands. The second paragraph of this article highlights the recent progress concerning the synthesis of composition controlled alloy nanoparticles by laser ablation in liquids. Here, binary and ternary alloy nanoparticles with totally homogeneous elemental distribution could be fabricated and the composition of these particles closely resembled bulk implant material. Finally, the model AuAg was used to systematically evaluate composition related toxicological effects of alloy nanoparticles. Here Ag(+) ion release is identified as the most probable mechanism of toxicity when recent toxicological studies with gametes, mammalian cells and bacteria are considered.
Keywords: albumin; gold-silver; implant alloy; laser ablation; nickel-titanium; size control; wear debris.
Figures
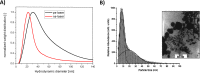
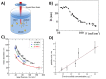
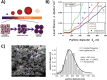
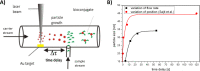
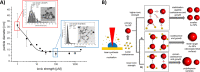
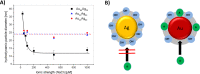
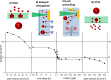
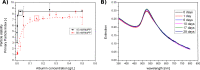
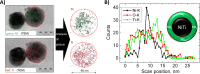
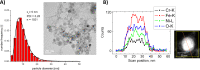
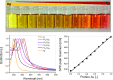
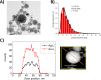
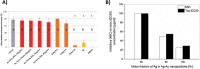
Similar articles
-
Monophasic ligand-free alloy nanoparticle synthesis determinants during pulsed laser ablation of bulk alloy and consolidated microparticles in water.Phys Chem Chem Phys. 2014 Nov 21;16(43):23671-8. doi: 10.1039/c4cp03316g. Epub 2014 Oct 1. Phys Chem Chem Phys. 2014. PMID: 25271711
-
Synthesis of Au-Ag Alloy Nanoparticles in Deionized Water by Pulsed Laser Ablation Technique.J Nanosci Nanotechnol. 2018 Jul 1;18(7):4841-4851. doi: 10.1166/jnn.2018.15358. J Nanosci Nanotechnol. 2018. PMID: 29442664
-
ns or fs pulsed laser ablation of a bulk InSb target in liquids for nanoparticles synthesis.J Colloid Interface Sci. 2016 May 1;469:57-62. doi: 10.1016/j.jcis.2016.01.069. Epub 2016 Jan 28. J Colloid Interface Sci. 2016. PMID: 26866890
-
Colloidal Metal Nanoparticles Prepared by Laser Ablation and their Applications.Chemphyschem. 2017 May 5;18(9):986-1006. doi: 10.1002/cphc.201601220. Epub 2017 Feb 27. Chemphyschem. 2017. PMID: 28164418 Review.
-
Progress in Laser Ablation and Biological Synthesis Processes: "Top-Down" and "Bottom-Up" Approaches for the Green Synthesis of Au/Ag Nanoparticles.Int J Mol Sci. 2022 Nov 24;23(23):14658. doi: 10.3390/ijms232314658. Int J Mol Sci. 2022. PMID: 36498986 Free PMC article. Review.
Cited by
-
Anti-biofilm properties of laser-synthesized, ultrapure silver-gold-alloy nanoparticles against Staphylococcus aureus.Sci Rep. 2024 Feb 10;14(1):3405. doi: 10.1038/s41598-024-53782-x. Sci Rep. 2024. PMID: 38336925 Free PMC article.
-
Unveiling Fundamentals of Multi-Beam Pulsed Laser Ablation in Liquids toward Scaling up Nanoparticle Production.Nanomaterials (Basel). 2024 Feb 16;14(4):365. doi: 10.3390/nano14040365. Nanomaterials (Basel). 2024. PMID: 38392738 Free PMC article.
-
Assessing the NLRP3 Inflammasome Activating Potential of a Large Panel of Micro- and Nanoplastics in THP-1 Cells.Biomolecules. 2022 Aug 9;12(8):1095. doi: 10.3390/biom12081095. Biomolecules. 2022. PMID: 36008988 Free PMC article.
-
Extensive Characterization of Oxide-Coated Colloidal Gold Nanoparticles Synthesized by Laser Ablation in Liquid.Materials (Basel). 2016 Sep 14;9(9):775. doi: 10.3390/ma9090775. Materials (Basel). 2016. PMID: 28773897 Free PMC article.
-
Solvent-surface interactions control the phase structure in laser-generated iron-gold core-shell nanoparticles.Sci Rep. 2016 Mar 23;6:23352. doi: 10.1038/srep23352. Sci Rep. 2016. PMID: 27004738 Free PMC article.
References
Publication types
LinkOut - more resources
Full Text Sources
Other Literature Sources