Structural and functional plasticity of astrocyte processes and dendritic spine interactions
- PMID: 25232111
- PMCID: PMC6705321
- DOI: 10.1523/JNEUROSCI.2401-14.2014
Structural and functional plasticity of astrocyte processes and dendritic spine interactions
Erratum in
- J Neurosci. 2014 Oct 15;34(42):14163
Abstract
Experience-dependent plasticity of synaptic transmission, which represents the cellular basis of learning, is accompanied by morphological changes in dendritic spines. Astrocytic processes are intimately associated with synapses, structurally enwrapping and functionally interacting with dendritic spines and synaptic terminals by responding to neurotransmitters and by releasing gliotransmitters that regulate synaptic function. While studies on structural synaptic plasticity have focused on neuronal elements, the structural-functional plasticity of astrocyte-neuron relationships remains poorly known. Here we show that stimuli inducing hippocampal synaptic LTP enhance the motility of synapse-associated astrocytic processes. This motility increase is relatively rapid, starting <5 min after the stimulus, and reaching a maximum in 20-30 min (t(1/2) = 10.7 min). It depends on presynaptic activity and requires G-protein-mediated Ca(2+) elevations in astrocytes. The structural remodeling is accompanied by changes in the ability of astrocytes to regulate synaptic transmission. Sensory stimuli that increase astrocyte Ca(2+) also induce similar plasticity in mouse somatosensory cortex in vivo. Therefore, structural relationships between astrocytic processes and dendritic spines undergo activity-dependent changes with metaplasticity consequences on synaptic regulation. These results reveal novel forms of synaptic plasticity based on structural-functional changes of astrocyte-neuron interactions.
Keywords: astrocyte; astrocyte–neuron interactions; dendritic spines; remodeling.
Copyright © 2014 the authors 0270-6474/14/3412738-07$15.00/0.
Figures
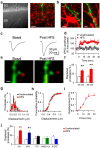
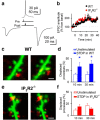
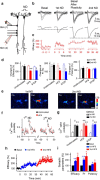
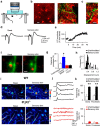
Similar articles
-
Cooperative astrocyte and dendritic spine dynamics at hippocampal excitatory synapses.J Neurosci. 2006 Aug 30;26(35):8881-91. doi: 10.1523/JNEUROSCI.1302-06.2006. J Neurosci. 2006. PMID: 16943543 Free PMC article.
-
Prolonged ampakine exposure prunes dendritic spines and increases presynaptic release probability for enhanced long-term potentiation in the hippocampus.Eur J Neurosci. 2014 Sep;40(5):2766-76. doi: 10.1111/ejn.12638. Epub 2014 Jun 13. Eur J Neurosci. 2014. PMID: 24925283
-
Functional Consequences of Synapse Remodeling Following Astrocyte-Specific Regulation of Ephrin-B1 in the Adult Hippocampus.J Neurosci. 2018 Jun 20;38(25):5710-5726. doi: 10.1523/JNEUROSCI.3618-17.2018. Epub 2018 May 23. J Neurosci. 2018. PMID: 29793972 Free PMC article.
-
Balancing structure and function at hippocampal dendritic spines.Annu Rev Neurosci. 2008;31:47-67. doi: 10.1146/annurev.neuro.31.060407.125646. Annu Rev Neurosci. 2008. PMID: 18284372 Free PMC article. Review.
-
Spine dynamics and synapse remodeling during LTP and memory processes.Prog Brain Res. 2008;169:199-207. doi: 10.1016/S0079-6123(07)00011-8. Prog Brain Res. 2008. PMID: 18394475 Review.
Cited by
-
DMV extrasynaptic NMDA receptors regulate caloric intake in rats.JCI Insight. 2021 May 10;6(9):e139785. doi: 10.1172/jci.insight.139785. JCI Insight. 2021. PMID: 33764905 Free PMC article.
-
Static magnetic fields reduce epileptiform activity in anesthetized rat and monkey.Sci Rep. 2018 Oct 30;8(1):15985. doi: 10.1038/s41598-018-33808-x. Sci Rep. 2018. PMID: 30375430 Free PMC article.
-
Pyridazine-derivatives Enhance Structural and Functional Plasticity of Tripartite Synapse Via Activation of Local Translation in Astrocytic Processes.Neuroscience. 2018 Sep 15;388:224-238. doi: 10.1016/j.neuroscience.2018.07.028. Epub 2018 Jul 27. Neuroscience. 2018. PMID: 30056115 Free PMC article.
-
The computational power of the human brain.Front Cell Neurosci. 2023 Aug 7;17:1220030. doi: 10.3389/fncel.2023.1220030. eCollection 2023. Front Cell Neurosci. 2023. PMID: 37608987 Free PMC article. Review.
-
TPC2-mediated Ca2+ signaling is required for the establishment of synchronized activity in developing zebrafish primary motor neurons.Dev Biol. 2018 Jun 1;438(1):57-68. doi: 10.1016/j.ydbio.2018.02.011. Epub 2018 Mar 23. Dev Biol. 2018. PMID: 29577882 Free PMC article.
References
Publication types
MeSH terms
Substances
LinkOut - more resources
Full Text Sources
Other Literature Sources
Molecular Biology Databases
Miscellaneous