Single-molecule analysis uncovers the difference between the kinetics of DNA decatenation by bacterial topoisomerases I and III
- PMID: 25232096
- PMCID: PMC4191389
- DOI: 10.1093/nar/gku785
Single-molecule analysis uncovers the difference between the kinetics of DNA decatenation by bacterial topoisomerases I and III
Abstract
Escherichia coli topoisomerases I and III can decatenate double-stranded DNA (dsDNA) molecules containing single-stranded DNA regions or nicks as well as relax negatively supercoiled DNA. Although the proteins share a mechanism of action and have similar structures, they participate in different cellular processes. Whereas topoisomerase III is a more efficient decatenase than topoisomerase I, the opposite is true for DNA relaxation. In order to investigate the differences in the mechanism of these two prototypical type IA topoisomerases, we studied DNA decatenation at the single-molecule level using braids of intact dsDNA and nicked dsDNA with bulges. We found that neither protein decatenates an intact DNA braid. In contrast, both enzymes exhibited robust decatenation activity on DNA braids with a bulge. The experiments reveal that a main difference between the unbraiding mechanisms of these topoisomerases lies in the pauses between decatenation cycles. Shorter pauses for topoisomerase III result in a higher decatenation rate. In addition, topoisomerase III shows a strong dependence on the crossover angle of the DNA strands. These real-time observations reveal the kinetic characteristics of the decatenation mechanism and help explain the differences between their activities.
© The Author(s) 2014. Published by Oxford University Press on behalf of Nucleic Acids Research.
Figures
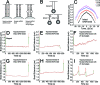




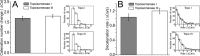
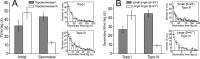
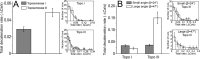
Similar articles
-
Bacterial topoisomerase I and topoisomerase III relax supercoiled DNA via distinct pathways.Nucleic Acids Res. 2012 Nov 1;40(20):10432-40. doi: 10.1093/nar/gks780. Epub 2012 Aug 25. Nucleic Acids Res. 2012. PMID: 22923519 Free PMC article.
-
Comparison of DNA decatenation by Escherichia coli topoisomerase IV and topoisomerase III: implications for non-equilibrium topology simplification.Nucleic Acids Res. 2013 Apr;41(8):4640-9. doi: 10.1093/nar/gkt136. Epub 2013 Mar 4. Nucleic Acids Res. 2013. PMID: 23460205 Free PMC article.
-
TopA, the Sulfolobus solfataricus topoisomerase III, is a decatenase.Nucleic Acids Res. 2018 Jan 25;46(2):861-872. doi: 10.1093/nar/gkx1247. Nucleic Acids Res. 2018. PMID: 29253195 Free PMC article.
-
Studies of bacterial topoisomerases I and III at the single-molecule level.Biochem Soc Trans. 2013 Apr;41(2):571-5. doi: 10.1042/BST20120297. Biochem Soc Trans. 2013. PMID: 23514156 Free PMC article. Review.
-
[DNA supercoiling and topoisomerases in Escherichia coli].Rev Latinoam Microbiol. 1995 Jul-Sep;37(3):291-304. Rev Latinoam Microbiol. 1995. PMID: 8850348 Review. Spanish.
Cited by
-
Jörg Langowski: his scientific legacy and the future it promises.BMC Biophys. 2018 Jul 16;11:5. doi: 10.1186/s13628-018-0045-1. eCollection 2018. BMC Biophys. 2018. PMID: 30026939 Free PMC article.
-
Unravelling the mechanisms of Type 1A topoisomerases using single-molecule approaches.Nucleic Acids Res. 2021 Jun 4;49(10):5470-5492. doi: 10.1093/nar/gkab239. Nucleic Acids Res. 2021. PMID: 33963870 Free PMC article. Review.
-
Microbial Type IA Topoisomerase C-Terminal Domain Sequence Motifs, Distribution and Combination.Int J Mol Sci. 2022 Aug 5;23(15):8709. doi: 10.3390/ijms23158709. Int J Mol Sci. 2022. PMID: 35955842 Free PMC article.
-
Torque and buckling in stretched intertwined double-helix DNAs.Phys Rev E. 2017 May;95(5-1):052401. doi: 10.1103/PhysRevE.95.052401. Epub 2017 May 1. Phys Rev E. 2017. PMID: 28618488 Free PMC article.
-
Variation of Structure and Cellular Functions of Type IA Topoisomerases across the Tree of Life.Cells. 2024 Mar 21;13(6):553. doi: 10.3390/cells13060553. Cells. 2024. PMID: 38534397 Free PMC article. Review.
References
-
- Schoeffler A.J., Berger J.M. DNA topoisomerases: harnessing and constraining energy to govern chromosome topology. Q. Rev. Biophys. 2008;41:41–101. - PubMed
-
- Forterre P., Gribaldo S., Gadelle D., Serre M.C. Origin and evolution of DNA topoisomerases. Biochimie. 2007;89:427–446. - PubMed
-
- Changela A., DiGate R.J., Mondragon A. Crystal structure of a complex of a type IA DNA topoisomerase with a single-stranded DNA molecule. Nature. 2001;411:1077–1081. - PubMed
-
- Li Z., Mondragon A., DiGate R.J. The mechanism of type IA topoisomerase-mediated DNA topological transformations. Mol. Cell. 2001;7:301–307. - PubMed
Publication types
MeSH terms
Substances
Grants and funding
LinkOut - more resources
Full Text Sources
Other Literature Sources
Molecular Biology Databases