Effects of human anti-spike protein receptor binding domain antibodies on severe acute respiratory syndrome coronavirus neutralization escape and fitness
- PMID: 25231316
- PMCID: PMC4248992
- DOI: 10.1128/JVI.02232-14
Effects of human anti-spike protein receptor binding domain antibodies on severe acute respiratory syndrome coronavirus neutralization escape and fitness
Abstract
The receptor binding domain (RBD) of the spike (S) glycoprotein of severe acute respiratory syndrome coronavirus (SARS-CoV) is a major target of protective immunity in vivo. Although a large number of neutralizing antibodies (nAbs) have been developed, it remains unclear if a single RBD-targeting nAb or two in combination can prevent neutralization escape and, if not, attenuate viral virulence in vivo. In this study, we used a large panel of human nAbs against an epitope that overlaps the interface between the RBD and its receptor, angiotensin-converting enzyme 2 (ACE2), to assess their cross-neutralization activities against a panel of human and zoonotic SARS-CoVs and neutralization escape mutants. We also investigated the neutralization escape profiles of these nAbs and evaluated their effects on receptor binding and virus fitness in vitro and in mice. We found that some nAbs had great potency and breadth in neutralizing multiple viral strains, including neutralization escape viruses derived from other nAbs; however, no single nAb or combination of two blocked neutralization escape. Interestingly, in mice the neutralization escape mutant viruses showed either attenuation (Urbani background) or increased virulence (GD03 background) consistent with the different binding affinities between their RBDs and the mouse ACE2. We conclude that using either single nAbs or dual nAb combinations to target a SARS-CoV RBD epitope that shows plasticity may have limitations for preventing neutralization escape during in vivo immunotherapy. However, RBD-directed nAbs may be useful for providing broad neutralization and prevention of escape variants when combined with other nAbs that target a second conserved epitope with less plasticity and more structural constraint.
Importance: The emergence of severe acute respiratory syndrome coronavirus (SARS-CoV) in 2002 and Middle East respiratory syndrome coronavirus (MERS-CoV) in 2012 has resulted in severe human respiratory disease with high death rates. Their zoonotic origins highlight the likelihood of reemergence or further evolution into novel human coronavirus pathogens. Broadly neutralizing antibodies (nAbs) that prevent infection of related viruses represent an important immunostrategy for combating coronavirus infections; however, for this strategy to succeed, it is essential to uncover nAb-mediated escape pathways and to pioneer strategies that prevent escape. Here, we used SARS-CoV as a research model and examined the escape pathways of broad nAbs that target the receptor binding domain (RBD) of the virus. We found that neither single nAbs nor two nAbs in combination blocked escape. Our results suggest that targeting conserved regions with less plasticity and more structural constraint rather than the SARS-CoV RBD-like region(s) should have broader utility for antibody-based immunotherapy.
Copyright © 2014, American Society for Microbiology. All Rights Reserved.
Figures
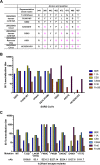
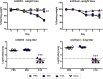
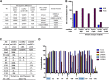
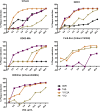
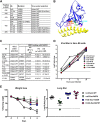
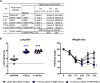
Similar articles
-
Recombinant Receptor-Binding Domains of Multiple Middle East Respiratory Syndrome Coronaviruses (MERS-CoVs) Induce Cross-Neutralizing Antibodies against Divergent Human and Camel MERS-CoVs and Antibody Escape Mutants.J Virol. 2016 Dec 16;91(1):e01651-16. doi: 10.1128/JVI.01651-16. Print 2017 Jan 1. J Virol. 2016. PMID: 27795425 Free PMC article.
-
Cross-neutralization of human and palm civet severe acute respiratory syndrome coronaviruses by antibodies targeting the receptor-binding domain of spike protein.J Immunol. 2006 May 15;176(10):6085-92. doi: 10.4049/jimmunol.176.10.6085. J Immunol. 2006. PMID: 16670317
-
Key residues of the receptor binding motif in the spike protein of SARS-CoV-2 that interact with ACE2 and neutralizing antibodies.Cell Mol Immunol. 2020 Jun;17(6):621-630. doi: 10.1038/s41423-020-0458-z. Epub 2020 May 15. Cell Mol Immunol. 2020. PMID: 32415260 Free PMC article.
-
Structural and antigenic variations in the spike protein of emerging SARS-CoV-2 variants.PLoS Pathog. 2022 Feb 17;18(2):e1010260. doi: 10.1371/journal.ppat.1010260. eCollection 2022 Feb. PLoS Pathog. 2022. PMID: 35176090 Free PMC article. Review.
-
Receptor-binding domain-specific human neutralizing monoclonal antibodies against SARS-CoV and SARS-CoV-2.Signal Transduct Target Ther. 2020 Sep 22;5(1):212. doi: 10.1038/s41392-020-00318-0. Signal Transduct Target Ther. 2020. PMID: 32963228 Free PMC article. Review.
Cited by
-
Development of a scalable single process for producing SARS-CoV-2 RBD monomer and dimer vaccine antigens.Front Bioeng Biotechnol. 2023 Nov 17;11:1287551. doi: 10.3389/fbioe.2023.1287551. eCollection 2023. Front Bioeng Biotechnol. 2023. PMID: 38050488 Free PMC article.
-
Cell entry mechanisms of SARS-CoV-2.Proc Natl Acad Sci U S A. 2020 May 26;117(21):11727-11734. doi: 10.1073/pnas.2003138117. Epub 2020 May 6. Proc Natl Acad Sci U S A. 2020. PMID: 32376634 Free PMC article.
-
SARS-like WIV1-CoV poised for human emergence.Proc Natl Acad Sci U S A. 2016 Mar 15;113(11):3048-53. doi: 10.1073/pnas.1517719113. Epub 2016 Mar 14. Proc Natl Acad Sci U S A. 2016. PMID: 26976607 Free PMC article.
-
Engineered ACE2 receptor traps potently neutralize SARS-CoV-2.Proc Natl Acad Sci U S A. 2020 Nov 10;117(45):28046-28055. doi: 10.1073/pnas.2016093117. Epub 2020 Oct 22. Proc Natl Acad Sci U S A. 2020. PMID: 33093202 Free PMC article.
-
Molecular Basis of Coronavirus Virulence and Vaccine Development.Adv Virus Res. 2016;96:245-286. doi: 10.1016/bs.aivir.2016.08.003. Epub 2016 Aug 30. Adv Virus Res. 2016. PMID: 27712626 Free PMC article. Review.
References
-
- Ksiazek TG, Erdman D, Goldsmith CS, Zaki SR, Peret T, Emery S, Tong S, Urbani C, Comer JA, Lim W, Rollin PE, Dowell SF, Ling AE, Humphrey CD, Shieh WJ, Guarner J, Paddock CD, Rota P, Fields B, DeRisi J, Yang JY, Cox N, Hughes JM, LeDuc JW, Bellini WJ, Anderson LJ. 2003. A novel coronavirus associated with severe acute respiratory syndrome. N. Engl. J. Med. 348:1953–1966. 10.1056/NEJMoa030781. - DOI - PubMed
-
- Liang G, Chen Q, Xu J, Liu Y, Lim W, Peiris JS, Anderson LJ, Ruan L, Li H, Kan B, Di B, Cheng P, Chan KH, Erdman DD, Gu S, Yan X, Liang W, Zhou D, Haynes L, Duan S, Zhang X, Zheng H, Gao Y, Tong S, Li D, Fang L, Qin P, Xu W. 2004. Laboratory diagnosis of four recent sporadic cases of community-acquired SARS, Guangdong Province, China. Emerg. Infect. Dis. 10:1774–1781. 10.3201/eid1010.040445. - DOI - PMC - PubMed
-
- Marra MA, Jones SJ, Astell CR, Holt RA, Brooks-Wilson A, Butterfield YS, Khattra J, Asano JK, Barber SA, Chan SY, Cloutier A, Coughlin SM, Freeman D, Girn N, Griffith OL, Leach SR, Mayo M, McDonald H, Montgomery SB, Pandoh PK, Petrescu AS, Robertson AG, Schein JE, Siddiqui A, Smailus DE, Stott JM, Yang GS, Plummer F, Andonov A, Artsob H, Bastien N, Bernard K, Booth TF, Bowness D, Czub M, Drebot M, Fernando L, Flick R, Garbutt M, Gray M, Grolla A, Jones S, Feldmann H, Meyers A, Kabani A, Li Y, Normand S, Stroher U, Tipples GA, Tyler S, Vogrig R, Ward D, Watson B, Brunham RC, Krajden M, Petric M, Skowronski DM, Upton C, Roper RL. 2003. The genome sequence of the SARS-associated coronavirus. Science 300:1399–1404. 10.1126/science.1085953. - DOI - PubMed
-
- Rota PA, Oberste MS, Monroe SS, Nix WA, Campagnoli R, Icenogle JP, Penaranda S, Bankamp B, Maher K, Chen MH, Tong S, Tamin A, Lowe L, Frace M, DeRisi JL, Chen Q, Wang D, Erdman DD, Peret TCT, Burns C, Ksiazek TG, Rollin PE, Sanchez A, Liffick S, Holloway B, Limor J, McCaustland K, Olsen-Rasmussen M, Fouchier R, Gunther S, Osterhaus ADME, Drosten C, Pallansch MA, Anderson LJ, Bellini WJ. 2003. Characterization of a novel coronavirus associated with severe acute respiratory syndrome. Science 300:1394–1399. 10.1126/science.1085952. - DOI - PubMed
Publication types
MeSH terms
Substances
Grants and funding
LinkOut - more resources
Full Text Sources
Other Literature Sources
Miscellaneous