An ankyrin repeat domain of AKR2 drives chloroplast targeting through coincident binding of two chloroplast lipids
- PMID: 25203210
- PMCID: PMC4170656
- DOI: 10.1016/j.devcel.2014.07.026
An ankyrin repeat domain of AKR2 drives chloroplast targeting through coincident binding of two chloroplast lipids
Abstract
In organellogenesis of the chloroplast from endosymbiotic cyanobacteria, the establishment of protein-targeting mechanisms to the chloroplast should have been pivotal. However, it is still mysterious how these mechanisms were established and how they work in plant cells. Here we show that AKR2A, the cytosolic targeting factor for chloroplast outer membrane (COM) proteins, evolved from the ankyrin repeat domain (ARD) of the host cell by stepwise extensions of its N-terminal domain and that two lipids, monogalactosyldiacylglycerol (MGDG) and phosphatidylglycerol (PG), of the endosymbiont were selected to function as the AKR2A receptor. Structural analysis, molecular modeling, and mutational analysis of the ARD identified two adjacent sites for coincidental and synergistic binding of MGDG and PG. Based on these findings, we propose that the targeting mechanism of COM proteins was established using components from both the endosymbiont and host cell through a modification of the protein-protein-interacting ARD into a lipid binding domain.
Copyright © 2014 Elsevier Inc. All rights reserved.
Figures
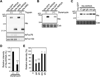
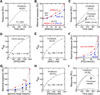
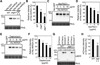
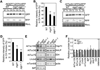
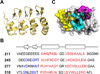
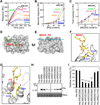
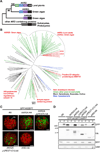
Comment in
-
Lipids guide the way: targeting proteins to the chloroplast outer envelope membrane.Dev Cell. 2014 Sep 8;30(5):493-5. doi: 10.1016/j.devcel.2014.08.017. Dev Cell. 2014. PMID: 25203205
Similar articles
-
Lipids guide the way: targeting proteins to the chloroplast outer envelope membrane.Dev Cell. 2014 Sep 8;30(5):493-5. doi: 10.1016/j.devcel.2014.08.017. Dev Cell. 2014. PMID: 25203205
-
Small heat shock protein Hsp17.8 functions as an AKR2A cofactor in the targeting of chloroplast outer membrane proteins in Arabidopsis.Plant Physiol. 2011 Sep;157(1):132-46. doi: 10.1104/pp.111.178681. Epub 2011 Jul 5. Plant Physiol. 2011. PMID: 21730198 Free PMC article.
-
Cytosolic targeting factor AKR2A captures chloroplast outer membrane-localized client proteins at the ribosome during translation.Nat Commun. 2015 Apr 16;6:6843. doi: 10.1038/ncomms7843. Nat Commun. 2015. PMID: 25880450
-
Head-Group Acylation of Chloroplast Membrane Lipids.Molecules. 2021 Feb 26;26(5):1273. doi: 10.3390/molecules26051273. Molecules. 2021. PMID: 33652855 Free PMC article. Review.
-
Role of Lipids in Chloroplast Biogenesis.Subcell Biochem. 2016;86:103-25. doi: 10.1007/978-3-319-25979-6_5. Subcell Biochem. 2016. PMID: 27023233 Review.
Cited by
-
Specific degradation of phosphatidylglycerol is necessary for proper mitochondrial morphology and function.Biochim Biophys Acta. 2016 Jan;1857(1):34-45. doi: 10.1016/j.bbabio.2015.10.004. Epub 2015 Oct 19. Biochim Biophys Acta. 2016. PMID: 26482708 Free PMC article.
-
TRPA1 ankyrin repeat six interacts with a small molecule inhibitor chemotype.Proc Natl Acad Sci U S A. 2018 Nov 27;115(48):12301-12306. doi: 10.1073/pnas.1808142115. Epub 2018 Nov 14. Proc Natl Acad Sci U S A. 2018. PMID: 30429323 Free PMC article.
-
Evolution of protein transport to the chloroplast envelope membranes.Photosynth Res. 2018 Dec;138(3):315-326. doi: 10.1007/s11120-018-0540-x. Epub 2018 Oct 5. Photosynth Res. 2018. PMID: 30291507 Review.
-
The decreased PG content of pgp1 inhibits PSI photochemistry and limits reaction center and light-harvesting polypeptide accumulation in response to cold acclimation.Planta. 2022 Jan 11;255(2):36. doi: 10.1007/s00425-022-03819-0. Planta. 2022. PMID: 35015152
-
Temperature-induced changes in the wheat phosphoproteome reveal temperature-regulated interconversion of phosphoforms.J Exp Bot. 2018 Aug 31;69(19):4609-4624. doi: 10.1093/jxb/ery204. J Exp Bot. 2018. PMID: 29939309 Free PMC article.
References
-
- Adams PD, Afonine PV, Bunkóczi G, Chen VB, Davis IW, Echols N, Headd JJ, Hung LW, Kapral GJ, Grosse-Kunstleve RW, McCoy AJ, Moriarty NW, Oeffner R, Read RJ, Richardson DC, Richardson JS, Terwilliger TC, Zwart PH. PHENIX: a comprehensive Python-based system for macromolecular structure solution. Acta. Crystallogr D. 2010;66:213–221. - PMC - PubMed
-
- Ananthanarayanan B, Stahelin RV, Digman MA, Cho W. Activation mechanisms of conventional protein kinase C isoforms are determined by the ligand affinity and conformational flexibility of their C1 domains. J. Biol. Chem. 2003;278:46886–46894. - PubMed
-
- Babiychuk E, Müller F, Eubel H, Braun HP, Frentzen M, Kushnir S. Arabidopsis phosphatidylglycerophosphate synthases 1 is essential for chloroplast differentiation, but is dispensable for mitochondrial function. Plant J. 2003;33:899–909. - PubMed
-
- Bae W, Lee YJ, Kim DH, Lee J, Kim S, Sohn EJ, Hwang I. AKR2A-mediated import of chloroplast outer membrane proteins is essential for chloroplast biogenesis. Nature Cell Biol. 2008;10:220–227. - PubMed
Publication types
MeSH terms
Substances
Grants and funding
LinkOut - more resources
Full Text Sources
Other Literature Sources
Molecular Biology Databases