The LIMP-2/SCARB2 binding motif on acid β-glucosidase: basic and applied implications for Gaucher disease and associated neurodegenerative diseases
- PMID: 25202012
- PMCID: PMC4208013
- DOI: 10.1074/jbc.M114.593616
The LIMP-2/SCARB2 binding motif on acid β-glucosidase: basic and applied implications for Gaucher disease and associated neurodegenerative diseases
Abstract
The acid β-glucosidase (glucocerbrosidase (GCase)) binding sequence to LIMP-2 (lysosomal integral membrane protein 2), the receptor for intracellular GCase trafficking to the lysosome, has been identified. Heterologous expression of deletion constructs, the available GCase crystal structures, and binding and co-localization of identified peptides or mutant GCases were used to identify and characterize a highly conserved 11-amino acid sequence, DSPIIVDITKD, within human GCase. The binding to LIMP-2 is not dependent upon a single amino acid, but the interactions of GCase with LIMP-2 are heavily influenced by Asp(399) and the di-isoleucines, Ile(402) and Ile(403). A single alanine substitution at any of these decreases GCase binding to LIMP-2 and alters its pH-dependent binding as well as diminishing the trafficking of GCase to the lysosome and significantly increasing GCase secretion. Enterovirus 71 also binds to LIMP-2 (also known as SCARB2) on the external surface of the plasma membrane. However, the LIMP-2/SCARB2 binding sequences for enterovirus 71 and GCase are not similar, indicating that LIMP-2/SCARB2 may have multiple or overlapping binding sites with differing specificities. These findings have therapeutic implications for the production of GCase and the distribution of this enzyme that is delivered to various organs.
Keywords: Enterovirus 71; Enzyme Kinetics; Glucocerebrosidase; Inborn Error of Metabolism; Intracellular Trafficking; Lysosomal Storage Disease; Lysosomal Targeting; Protein Sorting.
© 2014 by The American Society for Biochemistry and Molecular Biology, Inc.
Figures
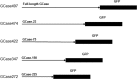
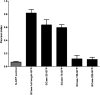
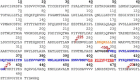
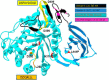
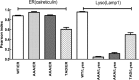
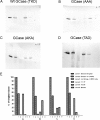
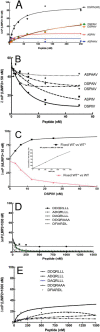
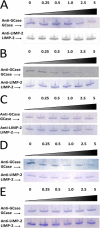
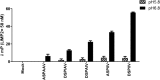
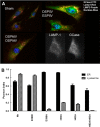
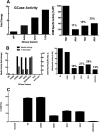
Similar articles
-
Lysosome sorting of β-glucocerebrosidase by LIMP-2 is targeted by the mannose 6-phosphate receptor.Nat Commun. 2014 Jul 14;5:4321. doi: 10.1038/ncomms5321. Nat Commun. 2014. PMID: 25027712 Free PMC article.
-
Deficiency of Glucocerebrosidase Activity beyond Gaucher Disease: PSAP and LIMP-2 Dysfunctions.Int J Mol Sci. 2024 Jun 16;25(12):6615. doi: 10.3390/ijms25126615. Int J Mol Sci. 2024. PMID: 38928321 Free PMC article.
-
Role of LIMP-2 in the intracellular trafficking of β-glucosidase in different human cellular models.FASEB J. 2015 Sep;29(9):3839-52. doi: 10.1096/fj.15-271148. Epub 2015 May 27. FASEB J. 2015. PMID: 26018676
-
Lysosomal integral membrane protein-2: a new player in lysosome-related pathology.Mol Genet Metab. 2014 Feb;111(2):84-91. doi: 10.1016/j.ymgme.2013.12.005. Epub 2013 Dec 11. Mol Genet Metab. 2014. PMID: 24389070 Free PMC article. Review.
-
Molecular regulations and therapeutic targets of Gaucher disease.Cytokine Growth Factor Rev. 2018 Jun;41:65-74. doi: 10.1016/j.cytogfr.2018.04.003. Epub 2018 Apr 11. Cytokine Growth Factor Rev. 2018. PMID: 29699937 Free PMC article. Review.
Cited by
-
Characterization of the complex formed by β-glucocerebrosidase and the lysosomal integral membrane protein type-2.Proc Natl Acad Sci U S A. 2016 Apr 5;113(14):3791-6. doi: 10.1073/pnas.1514005113. Epub 2016 Mar 21. Proc Natl Acad Sci U S A. 2016. PMID: 27001828 Free PMC article.
-
Mass spectrometry-based proteomics in neurodegenerative lysosomal storage disorders.Mol Omics. 2022 May 11;18(4):256-278. doi: 10.1039/d2mo00004k. Mol Omics. 2022. PMID: 35343995 Free PMC article. Review.
-
Standard-flow LC and thermal focusing ESI elucidates altered liver proteins in late stage Niemann-Pick, type C1 disease.Bioanalysis. 2019 Jun;11(11):1067-1083. doi: 10.4155/bio-2018-0232. Bioanalysis. 2019. PMID: 31251104 Free PMC article.
-
The Link between Gaucher Disease and Parkinson's Disease Sheds Light on Old and Novel Disorders of Sphingolipid Metabolism.Int J Mol Sci. 2019 Jul 5;20(13):3304. doi: 10.3390/ijms20133304. Int J Mol Sci. 2019. PMID: 31284408 Free PMC article. Review.
-
Impaired autophagy: The collateral damage of lysosomal storage disorders.EBioMedicine. 2021 Jan;63:103166. doi: 10.1016/j.ebiom.2020.103166. Epub 2020 Dec 17. EBioMedicine. 2021. PMID: 33341443 Free PMC article. Review.
References
-
- Brady R. O., Kanfer J. N., Shapiro D. (1965) Metabolism of glucocerebrosides. II. Evidence of an enzymatic deficiency in Gaucher's disease. Biochem. Biophys. Res. Commun. 18, 221–225 - PubMed
-
- Grabowski G. A., Petsko G. A., Kolodny E. (2010) Gaucher disease. in The Metabolic and Molecular Bases of Inherited Disease (Valle D., Beaudet A. L., Vogelstein B., Kinzler K. W., Antonarakis S. E., Ballabio A., Sly W. S., eds) McGraw-Hill, New York
-
- Grabowski G. A., Kolodny E. H., Weinreb N. J., Rosenbloom B. E., Prakash-Cheng A., Kaplan P., Charrow J., Pastores G. M., Mistry P. K. (2006) Gaucher disease: phenotypic and genetic variation. in The Metabolic and Molecular Bases of Inherited Diseases (Scriver C., Beaudet A., Sly W., Valle D., eds) McGraw-Hill, New York
-
- Liou B., Kazimierczuk A., Zhang M., Scott C. R., Hegde R. S., Grabowski G. A. (2006) Analyses of variant acid β-glucosidases: effects of Gaucher disease mutations. J. Biol. Chem. 281, 4242–4253 - PubMed
Publication types
MeSH terms
Substances
Grants and funding
LinkOut - more resources
Full Text Sources
Other Literature Sources
Medical
Molecular Biology Databases
Miscellaneous