Regulation of microtubule-based transport by MAP4
- PMID: 25143402
- PMCID: PMC4196864
- DOI: 10.1091/mbc.E14-01-0022
Regulation of microtubule-based transport by MAP4
Abstract
Microtubule (MT)-based transport of organelles driven by the opposing MT motors kinesins and dynein is tightly regulated in cells, but the underlying molecular mechanisms remain largely unknown. Here we tested the regulation of MT transport by the ubiquitous protein MAP4 using Xenopus melanophores as an experimental system. In these cells, pigment granules (melanosomes) move along MTs to the cell center (aggregation) or to the periphery (dispersion) by means of cytoplasmic dynein and kinesin-2, respectively. We found that aggregation signals induced phosphorylation of threonine residues in the MT-binding domain of the Xenopus MAP4 (XMAP4), thus decreasing binding of this protein to MTs. Overexpression of XMAP4 inhibited pigment aggregation by shortening dynein-dependent MT runs of melanosomes, whereas removal of XMAP4 from MTs reduced the length of kinesin-2-dependent runs and suppressed pigment dispersion. We hypothesize that binding of XMAP4 to MTs negatively regulates dynein-dependent movement of melanosomes and positively regulates kinesin-2-based movement. Phosphorylation during pigment aggregation reduces binding of XMAP4 to MTs, thus increasing dynein-dependent and decreasing kinesin-2-dependent motility of melanosomes, which stimulates their accumulation in the cell center, whereas dephosphorylation of XMAP4 during dispersion has an opposite effect.
© 2014 Semenova et al. This article is distributed by The American Society for Cell Biology under license from the author(s). Two months after publication it is available to the public under an Attribution–Noncommercial–Share Alike 3.0 Unported Creative Commons License (http://creativecommons.org/licenses/by-nc-sa/3.0).
Figures
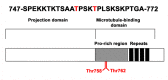
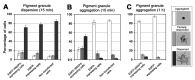
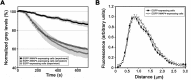
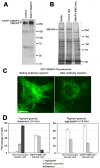
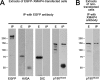
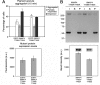
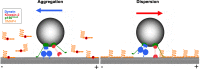
Similar articles
-
Dynein, dynactin, and kinesin II's interaction with microtubules is regulated during bidirectional organelle transport.J Cell Biol. 2000 Oct 2;151(1):155-66. doi: 10.1083/jcb.151.1.155. J Cell Biol. 2000. Retraction in: J Cell Biol. 2003 Feb 17;160(4):617. doi: 10.1083/jcb.20030203151155 PMID: 11018061 Free PMC article. Retracted.
-
Organelle transport along microtubules in Xenopus melanophores: evidence for cooperation between multiple motors.Biophys J. 2006 Jan 1;90(1):318-27. doi: 10.1529/biophysj.105.067843. Epub 2005 Oct 7. Biophys J. 2006. PMID: 16214870 Free PMC article.
-
CK1 activates minus-end-directed transport of membrane organelles along microtubules.Mol Biol Cell. 2011 Apr 15;22(8):1321-9. doi: 10.1091/mbc.E10-09-0741. Epub 2011 Feb 9. Mol Biol Cell. 2011. PMID: 21307338 Free PMC article.
-
Molecular mechanisms of pigment transport in melanophores.Pigment Cell Res. 1999 Oct;12(5):283-94. doi: 10.1111/j.1600-0749.1999.tb00762.x. Pigment Cell Res. 1999. PMID: 10541038 Review.
-
Melatonin, melatonin receptors and melanophores: a moving story.Pigment Cell Res. 2004 Oct;17(5):454-60. doi: 10.1111/j.1600-0749.2004.00185.x. Pigment Cell Res. 2004. PMID: 15357831 Review.
Cited by
-
MAP4 as a New Candidate in Cardiovascular Disease.Front Physiol. 2020 Aug 26;11:1044. doi: 10.3389/fphys.2020.01044. eCollection 2020. Front Physiol. 2020. PMID: 32982783 Free PMC article. Review.
-
Intracellular Cargo Transport by Kinesin-3 Motors.Biochemistry (Mosc). 2017 Jul;82(7):803-815. doi: 10.1134/S0006297917070057. Biochemistry (Mosc). 2017. PMID: 28918744 Free PMC article. Review.
-
MAP7 regulates organelle transport by recruiting kinesin-1 to microtubules.J Biol Chem. 2019 Jun 28;294(26):10160-10171. doi: 10.1074/jbc.RA119.008052. Epub 2019 May 13. J Biol Chem. 2019. PMID: 31085585 Free PMC article.
-
Phosphatidylinositol-3-OH kinase signalling is spatially organized at endosomal compartments by microtubule-associated protein 4.Nat Cell Biol. 2020 Nov;22(11):1357-1370. doi: 10.1038/s41556-020-00596-4. Epub 2020 Nov 2. Nat Cell Biol. 2020. PMID: 33139939 Free PMC article.
-
Kinesin-4 KIF21B limits microtubule growth to allow rapid centrosome polarization in T cells.Elife. 2020 Dec 21;9:e62876. doi: 10.7554/eLife.62876. Elife. 2020. PMID: 33346730 Free PMC article.
References
-
- Aizawa H, Emori Y, Mori A, Murofushi H, Sakai H, Suzuki K. Functional analyses of the domain structure of microtubule-associated protein-4 (MAP-U) J Biol Chem. 1991;266:9841–9846. - PubMed
-
- Aizawa H, Emori Y, Murofushi H, Kawasaki H, Sakai H, Suzuki K. Molecular cloning of a ubiquitously distributed microtubule-associated protein with Mr 190,000. J Biol Chem. 1990;265:13849–13855. - PubMed
-
- Aspengren S, Hedberg D, Skold HN, Wallin M. New insights into melanosome transport in vertebrate pigment cells. Int Rev Cell Mol Biol. 2009;272:245–302. - PubMed
-
- Atherton J, Houdusse A, Moores C. MAPping out distribution routes for kinesin couriers. Biol Cell. 2013;105:465–487. - PubMed
Publication types
MeSH terms
Substances
Grants and funding
LinkOut - more resources
Full Text Sources
Other Literature Sources