Challenges associated with Penetration of Nanoparticles across Cell and Tissue Barriers: A Review of Current Status and Future Prospects
- PMID: 25132862
- PMCID: PMC4129396
- DOI: 10.1016/j.nantod.2014.04.008
Challenges associated with Penetration of Nanoparticles across Cell and Tissue Barriers: A Review of Current Status and Future Prospects
Abstract
Nanoparticles (NPs) have emerged as an effective modality for the treatment of various diseases including cancer, cardiovascular and inflammatory diseases. Various forms of NPs including liposomes, polymer particles, micelles, dendrimers, quantum dots, gold NPs and carbon nanotubes have been synthesized and tested for therapeutic applications. One of the greatest challenges that limit the success of NPs is their ability to reach the therapeutic site at necessary doses while minimizing accumulation at undesired sites. The biodistribution of NPs is determined by body's biological barriers that manifest in several distinct ways. For intravascular delivery of NPs, the barrier manifests in the form of: (i) immune clearance in the liver and spleen, (ii) permeation across the endothelium into target tissues, (iii) penetration through the tissue interstitium, (iv) endocytosis in target cells, (v) diffusion through cytoplasm and (vi) eventually entry into the nucleus, if required. Certain applications of NPs also rely on delivery through alternate routes including skin and mucosal membranes of the nose, lungs, intestine and vagina. In these cases, the diffusive resistance of these tissues poses a significant barrier to delivery. This review focuses on the current understanding of penetration of NPs through biological barriers. Emphasis is placed on transport barriers and not immunological barriers. The review also discusses design strategies for overcoming the barrier properties.
Figures
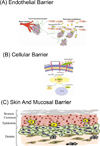
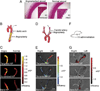
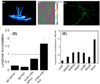
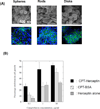
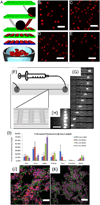
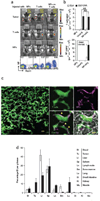
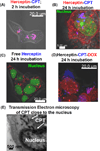
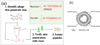
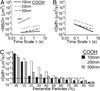
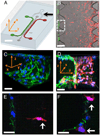
Similar articles
-
Theranostic Nanoparticles for RNA-Based Cancer Treatment.Acc Chem Res. 2019 Jun 18;52(6):1496-1506. doi: 10.1021/acs.accounts.9b00101. Epub 2019 May 28. Acc Chem Res. 2019. PMID: 31135134 Free PMC article. Review.
-
Tumor-Acidity-Cleavable Maleic Acid Amide (TACMAA): A Powerful Tool for Designing Smart Nanoparticles To Overcome Delivery Barriers in Cancer Nanomedicine.Acc Chem Res. 2018 Nov 20;51(11):2848-2856. doi: 10.1021/acs.accounts.8b00195. Epub 2018 Oct 15. Acc Chem Res. 2018. PMID: 30346728 Review.
-
Transport of PEGylated-PLA nanoparticles across a blood brain barrier model, entry into neuronal cells and in vivo brain bioavailability.J Control Release. 2020 Dec 10;328:679-695. doi: 10.1016/j.jconrel.2020.09.042. Epub 2020 Sep 23. J Control Release. 2020. PMID: 32979453
-
Convective diffusion of nanoparticles from the epithelial barrier toward regional lymph nodes.Adv Colloid Interface Sci. 2013 Nov;199-200:23-43. doi: 10.1016/j.cis.2013.06.002. Epub 2013 Jun 10. Adv Colloid Interface Sci. 2013. PMID: 23859221 Free PMC article. Review.
-
Strategies to improve tumor penetration of nanomedicines through nanoparticle design.Wiley Interdiscip Rev Nanomed Nanobiotechnol. 2019 Jan;11(1):e1519. doi: 10.1002/wnan.1519. Epub 2018 Apr 16. Wiley Interdiscip Rev Nanomed Nanobiotechnol. 2019. PMID: 29659166 Review.
Cited by
-
Reversible Treatment of Pressure Overload-Induced Left Ventricular Hypertrophy through Drd5 Nucleic Acid Delivery Mediated by Functional Polyaminoglycoside.Adv Sci (Weinh). 2021 Jan 6;8(5):2003706. doi: 10.1002/advs.202003706. eCollection 2021 Mar. Adv Sci (Weinh). 2021. PMID: 33717857 Free PMC article.
-
Hyaluronic Acid in the Intestinal Tract: Influence of Structure, Rheology, and Mucoadhesion on the Intestinal Uptake in Rats.Biomolecules. 2020 Oct 8;10(10):1422. doi: 10.3390/biom10101422. Biomolecules. 2020. PMID: 33050089 Free PMC article.
-
Therapeutic Strategies and Nano-Drug Delivery Applications in Management of Aging Alzheimer's Disease.Adv Exp Med Biol. 2021;1286:183-198. doi: 10.1007/978-3-030-55035-6_13. Adv Exp Med Biol. 2021. PMID: 33725354 Review.
-
A Hybrid In Silico and Tumor-on-a-Chip Approach to Model Targeted Protein Behavior in 3D Microenvironments.Cancers (Basel). 2021 May 18;13(10):2461. doi: 10.3390/cancers13102461. Cancers (Basel). 2021. PMID: 34070171 Free PMC article.
-
Nanocarriers for cancer nano-immunotherapy.Drug Deliv Transl Res. 2023 Jul;13(7):1936-1954. doi: 10.1007/s13346-022-01241-3. Epub 2022 Oct 3. Drug Deliv Transl Res. 2023. PMID: 36190661 Free PMC article. Review.
References
-
- Yoo JW, Chambers E, Mitragotri S. Curr. Pharm. Des. 2010;16:2298. - PubMed
-
- Galley HF, Webster NR. Br. J. Anaesth. 2004;93:105. - PubMed
-
- Jain RK. Cancer Res. 1987;47:3039. - PubMed
-
- Jain RK, Baxter LT. Cancer Res. 1988;48:7022. - PubMed
-
- Rakesh KJ, Triantafyllos S. Nat. Rev. Clin. Oncol. 2010;7:653. - PubMed
Grants and funding
LinkOut - more resources
Full Text Sources
Other Literature Sources