Neuronal hyperactivity recruits microglial processes via neuronal NMDA receptors and microglial P2Y12 receptors after status epilepticus
- PMID: 25100587
- PMCID: PMC4200107
- DOI: 10.1523/JNEUROSCI.0416-14.2014
Neuronal hyperactivity recruits microglial processes via neuronal NMDA receptors and microglial P2Y12 receptors after status epilepticus
Abstract
Microglia are highly dynamic immune cells of the CNS and their dynamism is proposed to be regulated by neuronal activities. However, the mechanisms underlying neuronal regulation of microglial dynamism have not been determined. Here, we found an increased number of microglial primary processes in the hippocampus during KA-induced seizure activity. Consistently, global glutamate induced robust microglial process extension toward neurons in both brain slices and in the intact brain in vivo. The mechanism of the glutamate-induced microglial process extension involves the activation of neuronal NMDA receptors, calcium influx, subsequent ATP release, and microglial response through P2Y12 receptors. Seizure-induced increases in microglial process numbers were also dependent on NMDA receptor activation. Finally, we found that P2Y12 KO mice exhibited reduced seizure-induced increases in microglial process numbers and worsened KA-induced seizure behaviors. Our results elucidate the molecular mechanisms underlying microglia-neuron communication that may be potentially neuroprotective in the epileptic brain.
Keywords: NMDA receptor; P2Y12 receptor; epilepsy; glutamate; microglia; process extension.
Copyright © 2014 the authors 0270-6474/14/3410528-13$15.00/0.
Figures
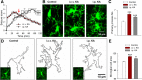
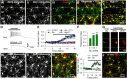
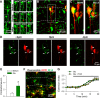
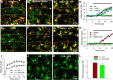
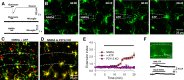
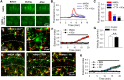
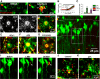
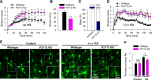
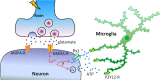
Similar articles
-
Activation of neuronal NMDA receptors triggers transient ATP-mediated microglial process outgrowth.J Neurosci. 2014 Aug 6;34(32):10511-27. doi: 10.1523/JNEUROSCI.0405-14.2014. J Neurosci. 2014. PMID: 25100586 Free PMC article.
-
Status epilepticus induces a particular microglial activation state characterized by enhanced purinergic signaling.J Neurosci. 2008 Sep 10;28(37):9133-44. doi: 10.1523/JNEUROSCI.1820-08.2008. J Neurosci. 2008. PMID: 18784294 Free PMC article.
-
Distinct P2Y Receptors Mediate Extension and Retraction of Microglial Processes in Epileptic and Peritumoral Human Tissue.J Neurosci. 2020 Feb 12;40(7):1373-1388. doi: 10.1523/JNEUROSCI.0218-19.2019. Epub 2020 Jan 2. J Neurosci. 2020. PMID: 31896671 Free PMC article.
-
Microglial circadian clock regulation of microglial structural complexity, dendritic spine density and inflammatory response.Neurochem Int. 2021 Jan;142:104905. doi: 10.1016/j.neuint.2020.104905. Epub 2020 Nov 18. Neurochem Int. 2021. PMID: 33217515 Review.
-
G-protein coupled purinergic P2Y12 receptor interacts and internalizes TauRD-mediated by membrane-associated actin cytoskeleton remodeling in microglia.Eur J Cell Biol. 2022 Apr;101(2):151201. doi: 10.1016/j.ejcb.2022.151201. Epub 2022 Jan 25. Eur J Cell Biol. 2022. PMID: 35101770 Review.
Cited by
-
Intracellular calcium dynamics in cortical microglia responding to focal laser injury in the PC::G5-tdT reporter mouse.Front Mol Neurosci. 2015 May 8;8:12. doi: 10.3389/fnmol.2015.00012. eCollection 2015. Front Mol Neurosci. 2015. PMID: 26005403 Free PMC article.
-
The immune system and metabolic products in epilepsy and glioma-associated epilepsy: emerging therapeutic directions.JCI Insight. 2024 Jan 9;9(1):e174753. doi: 10.1172/jci.insight.174753. JCI Insight. 2024. PMID: 38193532 Free PMC article. Review.
-
Role of glia in optic nerve.Prog Retin Eye Res. 2021 Mar;81:100886. doi: 10.1016/j.preteyeres.2020.100886. Epub 2020 Aug 6. Prog Retin Eye Res. 2021. PMID: 32771538 Free PMC article. Review.
-
Spinal microglia contribute to sustained inflammatory pain via amplifying neuronal activity.Mol Brain. 2022 Oct 26;15(1):86. doi: 10.1186/s13041-022-00970-3. Mol Brain. 2022. PMID: 36289499 Free PMC article.
-
Surveilling microglia dampens neuronal activity: operation of a purinergically mediated negative feedback mechanism.Signal Transduct Target Ther. 2021 Apr 17;6(1):160. doi: 10.1038/s41392-021-00586-4. Signal Transduct Target Ther. 2021. PMID: 33866328 Free PMC article. No abstract available.
References
-
- Butovsky O, Jedrychowski MP, Moore CS, Cialic R, Lanser AJ, Gabriely G, Koeglsperger T, Dake B, Wu PM, Doykan CE, Fanek Z, Liu L, Chen Z, Rothstein JD, Ransohoff RM, Gygi SP, Antel JP, Weiner HL. Identification of a unique TGF-beta-dependent molecular and functional signature in microglia. Nat Neurosci. 2014;17:131–143. doi: 10.1038/nn.3599. - DOI - PMC - PubMed
Publication types
MeSH terms
Substances
Grants and funding
LinkOut - more resources
Full Text Sources
Other Literature Sources
Molecular Biology Databases
Research Materials