The RNA binding of protein A from Wuhan nodavirus is mediated by mitochondrial membrane lipids
- PMID: 25092456
- PMCID: PMC7112130
- DOI: 10.1016/j.virol.2014.05.022
The RNA binding of protein A from Wuhan nodavirus is mediated by mitochondrial membrane lipids
Abstract
RNA replication of positive-strand (+)RNA viruses requires the lipids present in intracellular membranes, the sites of which viral replicases associate with. However, the direct effects of membrane lipids on viral replicases are still poorly understood. Wuhan nodavirus (WhNV) protein A, which associates with mitochondrial membranes, is the sole replicase required for RNA replication. Here, we report that WhNV protein A binds to RNA1 in a cooperative manner. Moreover, mitochondrial membrane lipids (MMLs) stimulated the RNA binding activity and cooperativity of protein A, and such stimulations exhibited strong selectivity for distinct phospholipids. Interestingly, MMLs stimulated the RNA-binding cooperativity only at higher protein A concentrations. Further investigation showed that MMLs stimulate the RNA binding of protein A by promoting its self-interaction. Finally, manipulating MML metabolism affected the protein A-induced RNA1 recruitment in cells. Together, our findings reveal the direct effects of membrane lipids on the RNA binding activity of a nodaviral replicase.
Keywords: Mitochondrial membrane lipids; Protein A; RNA binding; Wuhan Nodavirus.
Copyright © 2014 Elsevier Inc. All rights reserved.
Figures
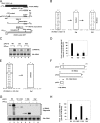
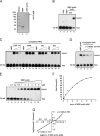
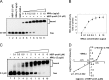
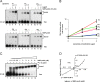
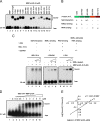
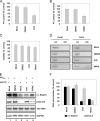
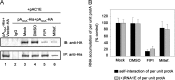
Similar articles
-
The self-interaction of a nodavirus replicase is enhanced by mitochondrial membrane lipids.PLoS One. 2014 Feb 25;9(2):e89628. doi: 10.1371/journal.pone.0089628. eCollection 2014. PLoS One. 2014. PMID: 24586921 Free PMC article.
-
Membrane association of Wuhan nodavirus protein A is required for its ability to accumulate genomic RNA1 template.Virology. 2013 May 10;439(2):140-51. doi: 10.1016/j.virol.2013.02.010. Epub 2013 Mar 11. Virology. 2013. PMID: 23490047
-
Characterization of a nodavirus replicase revealed a de novo initiation mechanism of RNA synthesis and terminal nucleotidyltransferase activity.J Biol Chem. 2013 Oct 25;288(43):30785-801. doi: 10.1074/jbc.M113.492728. Epub 2013 Sep 9. J Biol Chem. 2013. PMID: 24019510 Free PMC article.
-
Expanding use of multi-origin subcellular membranes by positive-strand RNA viruses during replication.Curr Opin Virol. 2014 Dec;9:119-26. doi: 10.1016/j.coviro.2014.09.015. Epub 2014 Oct 22. Curr Opin Virol. 2014. PMID: 25462443 Review.
-
Cell-Free and Cell-Based Approaches to Explore the Roles of Host Membranes and Lipids in the Formation of Viral Replication Compartment Induced by Tombusviruses.Viruses. 2016 Mar 3;8(3):68. doi: 10.3390/v8030068. Viruses. 2016. PMID: 26950140 Free PMC article. Review.
Cited by
-
Activation of Tomato Bushy Stunt Virus RNA-Dependent RNA Polymerase by Cellular Heat Shock Protein 70 Is Enhanced by Phospholipids In Vitro.J Virol. 2015 May;89(10):5714-23. doi: 10.1128/JVI.03711-14. Epub 2015 Mar 11. J Virol. 2015. PMID: 25762742 Free PMC article.
-
RNA chaperones encoded by RNA viruses.Virol Sin. 2015 Dec;30(6):401-9. doi: 10.1007/s12250-015-3676-2. Epub 2015 Dec 22. Virol Sin. 2015. PMID: 26715301 Free PMC article. Review.
References
-
- Arnold J.J., Cameron C.E. Poliovirus RNA-dependent RNA polymerase (3Dpol) is sufficient for template switching in vitro. J. Biol. Chem. 1999;274:2706–2716. - PubMed
Publication types
MeSH terms
Substances
LinkOut - more resources
Full Text Sources
Other Literature Sources